生命起源

古生物 |
古生物学 |
---|
![]() |
Portal:生物学 Category:古生物学 |


自然发生论,或者说生命起源[2][3][4][a],指的是自然历程中无生命物质如何演变为有机分子乃至生命的学说[5]:vi[3][6][7]:47。目前普遍接受的假设是,地球上从非生物到生物的过渡不是一个单一的事件,而是一个进化的过程(即一个复杂性逐渐增加的过程),其中涉及到宜居星球的形成、有机分子的前生物合成、可自我复制、自组装、自催化的分子,及细胞膜的出现。[8][9][10]对这一过程的不同阶段,存在许多假说。[11]
对生物起源的研究旨在确定前生命化学反应如何在与今日截然不同的环境下产生生命[12]:29。其主要使用生物学和化学理论为工具[13],最近的研究试图综合更多学科探讨这一问题。生命活动主要依托碳和水的专门化学反应,主要依赖4类主要的化学物质:用于构建细胞膜的脂质、糖类等碳水化合物、用于蛋白质代谢的氨基酸,以及用于遗传机制的核酸,分为DNA和RNA两种。任何成功的生物起源学说,都必须对这些分子类别的起源和相互作用加以解释[14]。许多研究生物起源的方法都在研究自我复制的分子或其组成部分是如何产生的。研究者们普遍认为,目前的生命来自RNA世界[15],不过在RNA诞生之前,可能已经存在别的可以自我复制的分子。
1952年经典的米勒-尤里实验证明,组成蛋白质的大多数氨基酸可以在复制了早期地球的环境下从无机化合物中合成出来。闪电、辐射、微陨石进入大气层产生的热量,以及海浪和洋流中的气泡内爆等外部能量来源可能引发了这些反应。其他假说(如“代谢在先”假说)则侧重于了解早期地球化学系统的催化作用如何产生了自我复制所需的前体分子[16]。
一种基因组学研究试图通过识别古菌和细菌(生物的两大主要分支,也称双域系统,其中真核生物属于古菌一支)共享的基因,来描述现代生物的最后共同祖先(LUCA)的基本特征。似乎有355个基因为所有生命共有,它们的性质表明LUCA可能是以伍德-隆达尔代谢途径进行代谢活动的厌氧生物,通过化学渗透获取能量,并以DNA为遗传密码,配以核糖体维持其遗传物质。尽管LUCA生存在距今40余亿年前,但研究者们并不认为它是生命的第一种形式。早期的细胞可能有一个渗漏膜(leaky membrane),并从海底热泉喷口附近自然产生的质子浓度梯度中获取能量。
迄今为止,地球仍是宇宙中唯一已知孕育了生命的地方[17][18]:xvii,地球上的化石证据为大多数关于生命起源的研究提供了参考。地球大约形成于45.4亿年前[19][20];地球上最早的无可争议的生命证据至少可以追溯到35亿年前[21][22][23]。加拿大魁北克努夫亚吉图克绿岩带的海底热泉沉淀物中发现了化石化了的微生物,这可能是地球上最古老的生命形式的推定证据,它们似乎在37.7-42.8亿年前生活在热液喷口沉积物中,这距离44亿年前冥古宙海洋形成并不久。[24]
概述
[编辑]
生命由带有(可遗传)变异的繁殖组成。[26]NASA将生命定义为“能进行达尔文进化的自我维持的化学系统”。[27]这样一个系统是复杂的;而最后共同祖先(LUCA)估计是生活在约40亿年前的单细胞生物,已经有数百个基因编码为今日普遍存在的DNA遗传码。这反过来又意味着LUCA一定有一套与DNA生物相似的细胞机器,如信使RNA、转运RNA、核糖体等,以按DNA编码组建蛋白质。这些蛋白质包括通过通过伍德-隆达尔代谢途径进行无氧呼吸的酶,以及复制遗传物质的DNA聚集酶。[28][9]
生命起源[29][3][4]研究者面临的挑战是,如何通过进化步骤解释这样一个复杂而又紧密联系的系统的产生,因为乍一看,它的每个部分都是能使它正常运转的必要条件。例如,一个细胞,无论是LUCA还是现代生命体,都会用DNA聚集酶复制其DNA,而DNA聚集酶又是通过翻译DNA中的DNA聚集酶基因产生的。酶和DNA的产生都离不开对方。[30]进化过程可能涉及分子的自我复制、细胞膜等部件的自组装和自催化反应。[28][9][10]
产生LUCA这样的活细胞的前提条件是很清楚的,尽管在细节上有争议:那大抵是一个有充足矿物和液态水供应的宜居环境。前生物合成创造了一系列简单的有机化合物,它们被组装成聚集物,如蛋白质和RNA。LUCA之后的过程也很好理解:生物进化创造了一系列具有不同形态和生化能力的物种。然而,像LUCA这样的生命体从无生命环境中衍生出来的过程还远未被理解。[1]
地球仍是已知唯一存在生命的地方,[17][18]:xvii而天体生物学试图寻找其他星球上的生命证据。2015年,NASA关于生命起源的战略旨在通过确定相互作用、中间结构和功能、能量来源和环境因素,来解释可进化大分子系统的多样性、选择和复制,[25]并绘制可能的原始信息聚集物的化学图景。它认为,能复制、储存遗传信息并表现出受选择的特性的聚集物,可能是前生物化学进化涌现的关键步骤之一。[25]这些聚集物又来自简单的有机化合物,如核碱基、氨基酸、糖,它们可能是由环境中的反应自然产生的。[5]:vi[3][31][32]任何成功的生物起源学说,都必须对所有这些化学物质的起源和相互作用加以解释。[33]
1960年代前的概念演变
[编辑]
自然发生
[编辑]从亚里士多德时代延续到19世纪的生命起源理论是自然发生:[34]“低等的”动物是由腐烂的有机物生成的,生命的产生是偶然的。[35]:229–258[36]托马斯·布朗的《常见错误(Pseudodoxia Epidemica)》(1646)中,对这一理论提出了质疑。[37][38]1665年,罗伯特·胡克发表了第一张微生物的图像。1676年,安东尼·范·列文胡克绘制并描述了微生物,可能是原生生物或细菌。[39]列文胡克不同意自然发生理论,1680年代,他进行了肉类在密封和开放环境下的肉类腐烂对照实验,以及对昆虫繁殖的仔细研究,证明了这一理论的不正确。[40]1668年,弗朗切斯科·雷迪指出,将肉置于苍蝇无法产卵的环境中时,肉不会生蛆[41]到19世纪中叶,自然发生理论基本不再是共识。[42]:196[43]
胚种论
[编辑]另一个可以追溯到公元前5世纪的古老思想是阿那克萨哥拉最先提出的胚种论[44],即认为生命存在于整个宇宙,由流星体、小行星、彗星[45]和太阳系小天体带到地球上。[46]它并不试图解释生命究竟如何起源,只是将生命的起源从地球上转移出去。这样做的好除上述,生命不需要在它出现的每颗行星上都形成一遍,而是在更有限的地点(甚至可能是单一地点)形成,然后通过彗星或陨石撞击,在银河系中传播。[47]
原始汤
[编辑]生命起源于非生命物质的缓慢阶段的观点见于赫伯特·斯潘塞《生物学原理》(1864–1867)、威廉·特纳·西塞尔顿-戴尔的论文《论自发产生和进化》(1879)。1871年2月1日,查尔斯·达尔文在给约瑟夫·道尔顿·胡克的信中谈到了这些出版物,并提出了自己的推测,认为生命的最初起源可能始于一个“温暖的小池塘,有氨和磷酸盐,外部有充足的光、热、电,化学反应产生了一种蛋白质化合物,并准备进行更复杂的变化……在今天,这种物质会被立即吞噬或吸收,生物出现以前则不会发生这种事。”[48][49][50]
亚历山大·伊万诺维奇·奥巴林(1924)和J. B. S. 霍尔丹(1929)先后提出,构成最早细胞的第一批分子是从原始汤中慢慢自我组织起来的,这一理论被称为奥巴林–霍尔丹假说。[51][52]霍尔丹提出,地球的前生物海洋基本是一大锅“热稀汤”,有机化合物可能形成于其中。[36][53]:300–302J·D·伯纳尔指出,这种机制可以从无机前体形成生命所需的大部分分子。[54]1967年,他提出了三个“阶段”:单体的起源;聚集物的起源;分子到细胞的演变。[55][56]
米勒-尤里实验
[编辑]1952年,斯坦利·米勒和哈罗德·尤里进行了一项化学实验,以验证有机分子如何在奥巴林–霍尔丹假说所假设的前生物条件下从无机前体中自发形成。实验使用了具有高还原性的气体混合物,其中包含甲烷、氨、氢及水蒸气,来形成简单的有机单体,如氨基酸。[57][58]贝尔纳(Bernal)在谈到米勒-尤里实验时说:“仅仅解释这种分子的形成是不够的,需要的是对这些分子的起源进行物理化学解释,表明存在合适的自由能来源。”[59]然而,目前的共识认为原始大气应具有弱还原性或中性,[60][61]这样大大限制了可能产生的氨基酸的数量和种类。然而,早期海洋中存在的铁和碳酸盐矿物的加入,产生了多样的氨基酸。[60]后来的研究工作关注另外两种潜在的还原性环境:外层空间和深海热液喷口。[62]:93–95[63]:56–57[64]
宜居地球的诞生
[编辑]拥有第一批恒星的早期宇宙
[编辑]宇宙大爆炸之后不久,宇宙中的化学元素只有氢、氦、锂,是最轻的3种原子。这些元素逐渐聚集在一起,形成了恒星。这些早期恒星质量大且寿命短,通过恒星核合成产生所有较重的元素。碳是宇宙中丰度第四多的元素(排在氢氦氧之后),主要形成于白矮星中,特别是那些大于太阳质量两倍的。[65]这些恒星的生命周期即将结束时,它们会将这些较重的元素(包括碳和氧)抛射到整个宇宙。这些较重的元素使得新的星体得以形成,包括岩质行星和其他天体。[66]根据星云假说,太阳系的形成与进化始于46亿年前,一片巨大分子云的一小部分发生了引力坍缩。坍缩物质的大部分质量聚集在中心,形成了太阳,而其余的则变扁平,形成原行星盘,其中诞生了行星、卫星、小行星和其他太阳系小天体。.[67]
地球的出现
[编辑]地球形成于约45.4亿年前。[19][68]:205–221冥古宙地球(自形成至40亿年前)起初不适宜任何生命生存。在其形成过程中,地球失去了其初始质量的很大一部分,因此缺乏容纳分子氢和大部分惰性气体的引力。[69]:9大气层主要由水蒸气、氮和二氧化碳组成,还有少量的一氧化碳、氢和硫化物。 [70]二氧化碳在水中的溶解,使得海洋呈微酸性,pH值约为5.5。[71]冥古宙大气被描述为一个“巨大、多产的户外化学实验室”,[72]类似于今日的火山气体,仍然支持一些非生物化学过程。[72]
海洋可能在地球形成后2亿年内就出现了,可能处于接近沸腾(100°C)的还原环境,pH值从5.8附近迅速上升到中性。[73]这一设想可从来自澳大利亚西部纳尔耶尔(Narryer)片麻岩地体的变质石英岩中形成于44.04亿年前的锆石结晶得到旁证。[74]火山活动可能有过显著增加,但在44-43亿年前,地球可能是一个水世界,几乎没有陆壳,水圈受到来自金牛T星阶段的太阳的紫外线、宇宙线辐射和持续的小行星和彗星撞击。[75]
后期重轰炸期假说认为,42.8亿年前[24]到38亿年前的宇宙环境对生命来说非常危险。按尼斯模型,巨行星轨道的变化可能使地球受到小行星和彗星的轰炸,并使月球和内行星遭受了巨大的破坏。[76]频繁的撞击会使得光合作用变得不可能。[72][77][78][79]这种破坏性事件的间隙,为早期环境中生命的可能起源提供了窗口期。如果生命源于深海热液环境,那么生命诞生的时间可能早在42-40亿年前。如果生命起源于陆地上,那么生命诞生的时间可能只发生在37-40亿年前。[80]然而,新的月面考察和样本使得连尼斯模型的倡导者都闭口不提后期重轰炸期的重要性。[81]
如果生命在十几米深的海洋中进化,上方的水就足够深,既不会使生命受到后期重轰炸的影响,也不会受当时太阳的强紫外线辐射影响。地热加热的洋壳可能通过深海热液喷口产生比米勒-尤里实验多得多的有机物。[82]:155可用的热量在100–150 °C时达到最大,这是超嗜热细菌和嗜热嗜酸古菌生活的温度。这些现代生物可能是最接近LUCA的现存生物。[83]
最早的生命证据
[编辑]生命在地球上已经绵延了超过35亿年,[21][22][23]至少在始太古代,地壳已经基本彻底凝固时就已经存在了。[84][85][86]迄今为止发现的最早的生命证据是加拿大魁北克努夫亚吉图克绿岩带的微生物化石,分散在年代介于37.7-42.8亿年前由海底热泉沉淀物形成的条状铁层中。这距离44亿年前海洋形成并不久。这些微生物与现代的热液喷口细菌相似,说明生命可以在这种环境中生存,自然也可能在这里产生。[24]
格陵兰西南37亿年前的变质沉积岩中发现了生物石墨。[87]格陵兰西南伊苏阿绿岩带附近的阿基利亚岛上,37亿年前的岩石中,发现了生物碳同位素特征。[82]伊苏阿绿岩带的其他部分,被困在石榴石晶体内的石墨包裹体,与生命的其他元素有关:氧、氮,可能还有磷酸盐形式的磷,为37亿年前的生命提供了进一步的证据。[88]澳大利亚西部34.9亿年前的砂岩中发现了菌毯化石[89]澳洲西部皮尔布拉地区,一片海滩化石的含黄铁矿砂岩中,发现了早期生命确凿的证据:圆管状的细胞,在没有氧气的情况下通过光合作用氧化硫。[90][91]来自澳洲西部的锆石暗示,地球至少在41亿年前就存在生命。[92]
澳洲西部皮尔布拉地区包含有34.8亿年前的德雷瑟(Dresser)组地层,包括被称为叠层石的分层结构。它们的现代对应物是包括蓝细菌在内的光合微生物。[93]它们位于未变形的热液沉积地层中,其纹理带有生物源特征。德雷瑟组地层的部分地区保留了陆地上的温泉,而其他地区似乎是浅海。[94]
产生分子:前生物合成
[编辑]除氢和氦之外的所有化学元素都来自恒星核合成。生命的基本化学成分——碳氢分子(CH)、碳氢正离子(CH+)和碳离子(C+)——是由恒星发出的紫外线形成的。[95]复杂的分子,包括有机分子,在太空和行星上都可以自然形成。[96]早期地球上的有机分子可能来自陆地,其合成过程是由撞击冲击或紫外线、氧化还原耦联或放电等其它能源驱动;也可能来自地外(伪泛生论),有机分子率先产生于星际云,后来降落到地球上。[97][98]
观测到的地外有机分子
[编辑]分子中含有碳的化学物质,绝大多数都是有机化合物。太阳、恒星、彗星和大多数行星的大气层中,碳都十分丰富。[99]有机化合物在太空中比较常见,由分子云和星周包层中“复杂的分子合成工厂”形成,并在反应开始后由游离辐射引发化学反应。[96][100][101]在陨石中发现了嘌呤和嘧啶核碱基,包括鸟嘌呤、腺嘌呤、胞嘧啶、尿嘧啶和胸腺嘧啶。这可能为DNA和RNA在早期地球上的形成提供了材料。[102]甘氨酸见于维尔特二号彗星喷出的物质,它早先曾在陨石中探测到。[103]彗星上包裹着的黑色物质,可能是由简单的碳化合物在电离辐射下形成的焦油状有机物。彗星的物质喷射可能把带有此类复杂分子的物质带到了地球上。[104][105][72]据估计,在后期重轰炸期期间,陨石每年可能向地球输送多达500万吨的前生物有机物。[72]
多环芳烃(PAH)世界假说
[编辑]
绿色区域是来自高温恒星的辐射与多环芳烃(PAH)组成的尘埃颗粒碰撞时发出的荧光。斯皮策空间望远镜摄于2018年
多环芳烃(PAH)是可观测宇宙中最常见、最丰富的多原子分子,是碳的主要存在形式。[99][106][107][108]它们似乎是在宇宙大爆炸后不久形成的,[109][107][108]并与新恒星和太阳系外行星有关。[99]它们很可能也是地球原始海洋的成分之一。[109][107][108]在星云、[110]星际物质、彗星和陨石中都检测到了多环芳烃。[99]
PAH世界假说认为,PAH是RNA世界的前身。[111]HH 46-IR是一颗类似于太阳早期的恒星,被一个物质盘包裹,其中的分子有氰化物、烃和一氧化碳。星际介质中的PAH可以通过氢化、氧化和羟基化变为活体细胞可用的更复杂的有机化合物。[112]
核碱基
[编辑]星际尘埃带到地球上的大多数有机化合物,由于其特殊的表面催化作用,有助于形成复杂的分子。[113][114]对默奇森陨石所带的有机化合物的12C/13C的同位素丰度的研究表明,RNA成分中的尿嘧啶和黄嘌呤等相关分子,是在地外形成的。[115]NASA对陨石的研究表明,所有4种DNA核碱基(腺嘌呤、鸟嘌呤和相关有机物)都是在外太空形成的。[113][116][117]宇宙尘埃含有复杂的有机物(“具有芳香性–脂肪族化合物混合结构的无定形有机固体”),可能由恒星迅速产生。[118]原恒星周围和陨石上可探测到糖醛,它是一种糖类分子,也是RNA前体的一种。[119][120]
实验室合成
[编辑]早在1860年代,实验就证明了,简单的碳源与丰富的无机催化剂的相互作用,足以产生生物大分子。但是,在原始汤理论所假设的条件下,从非生物生成的单体自发形成复杂的聚集物也不简单。在米勒-尤里实验和约安·奥罗的实验中,除了必要的有机单体之外,也形成了高浓度的阻止其他物质形成聚集物的物质。[121]蛋白质酶的编码中使用了约20种氨基酸,这是可能的产物中一个非常小的子集。生命倾向于使用任何可用的东西,所以为什么蛋白质编码只用了这样几种氨基酸需要合理的解释。[122]
糖类
[编辑]
布特列洛夫在1861年指出,当甲醛在碱性条件下与钙等二价金属离子一起加热时,聚糖反应就会产生包括四糖、五糖、六糖在内的糖类。布雷斯罗在1959年指出,该反应是自催化反应。[123]
核碱基
[编辑]像鸟嘌呤和腺嘌呤这样的核碱基可以从简单的碳、氮源(如氰化氢(HCN)和氨)中合成出来。[124]甲酰胺在与地球上的矿物一起加热时,可以产生所有4种核苷酸。甲酰胺在宇宙中无处不在,由水和HCN的反应产生。它可以通过水的蒸发来浓缩。[125][126]HCN只对当时还不存在的好氧生物(真核生物与需氧性细菌)有毒,还可以在甘氨酸的合成等化学过程中发挥作用。[72]
包括尿嘧啶、胞嘧啶和胸腺嘧啶在内的DNA和RNA成分可以在外太空中,利用陨石中发现的嘧啶等前体合成出来。嘧啶可能形成于红巨星、星际尘埃和气体云里。[127]所有四种RNA碱基可能是在高能量密度事件(如陨石撞击)中从甲酰胺合成的。[128]
其他从无机材料合成碱基的途径也有报道。[129]冻结温度对合成嘌呤是有利的,因为这对关键前体,如氰化氢有浓缩作用。[130]然而,胞嘧啶和尿嘧啶可能需要接近沸腾的温度。 [131]将氨和氰化物在冰柜中放置25年后,冰中形成了7种氨基酸和11种核碱基。[132][133]使尿素溶液在还原性环境下进行冻融循环,并以火花放电作为能量来源,可以合成出S-三嗪(代替核碱基)、嘧啶(包括胞嘧啶和尿嘧啶)和腺嘌呤。[134]对于在如此低的温度下这些反应的不寻常速度,普遍认同的解释是共晶冻结:杂质被挤在冰内的微型液袋中,分子会更频繁地碰撞。[135]
产生合适的囊泡
[编辑]脂质世界理论认为,第一个实现了自我复制的物体应类似于脂质。[136][137]磷脂在水中被搅动时,可能会形成磷脂双分子层——与细胞膜的结构相同。这些分子在早期地球上并不存在,但其他两亲分子链也会形成膜。插入额外的脂质可以扩展这些组织,并可能自发地分裂成两个大小、成分相似的后代。主要观点是,脂质体的分子组成是信息存储的第一步,像RNA这样的存储信息的聚集物是在后来的进化中出现的。对可能存在于前生物世界的两亲分子囊泡的研究,迄今只限于一两种两亲分子。[138]
脂质双层膜可以由大量两亲分子排列组合而成。其中最好的会有利于构成一个正反馈循环,[139][140]分别反映着一个膜位置和一个被困在囊泡中的特定化合物。这种位置-化合物对可以传递给子囊泡,导致囊泡出现不同品系,这将使自然选择成为可能。[141]
原初生命体应当是自组织的球形脂质集合体,是生命起源的垫脚石。[138]经典不可逆热力学理论在耗散系统的框架内,以广义的化学势处理自组装问题。[142][143][144]
进化中的一个核心问题是,简单的原生细胞最初究竟是如何出现的,还要在对下一代的繁殖贡献上有所差别,这样才能推动生命的进化。 截至2014年,功能性的原细胞尚未在实验室环境中实现。[145][146][147]自组装囊泡是原初生命体的重要组成部分。[138]热力学第二定律要求宇宙朝着熵增加的方向发展,但生命的特点是具有很大的组织度。因此,必须有一个边界隔开生命过程与无生命环境。[148]伊蕾娜·陈(Irene Chen)和杰克·绍斯塔克认为,基本的原生细胞可以产生细胞行为,包括原始形式的有差异繁殖、竞争和能量储存。[146]对膜分子的竞争将有利于稳定的膜的产生,这表明交联脂肪酸甚至今日的磷脂,在进化中具有选择优势。[146]这种微囊化将允许膜内进行新陈代谢和小分子的交换,同时将大的生物分子留在里面。细胞需要这种膜来创造自己的电化学梯度,以通过泵送离子过膜来储存能量。[149][150]
生成生物
[编辑]能量和熵
[编辑]当分子组织成生命物质时,生命需要排除熵。生命的出现和增加并不与热力学第二定律相矛盾,因为生命体在某些地方(如其体内)创造秩序,会以其他地方的熵增(如热量和废物的产生)为代价。[151][152][153]
早期地球上有许多能量来源可用于化学反应。比如来自地热过程的热量,便是化学的一个标准能源。其他例子包括阳光、闪电、[72]微陨石进入大气、[154]海洋和海浪中气泡的内爆等。[155]这已被实验[156][157]和模拟所证实。[158] 不利的反应可以由高度有利的反应驱动,如铁-硫反应。这可能大大促进了固碳。[b] 通过铁-硫反应,可以使CO2与H2S实现固碳,这在中性pH值和100°C的环境下尤为有利。热液喷口附近丰富的铁硫表面,可以推动少量氨基酸和其他生物大分子的生产。[72]
化学渗透
[编辑]
1961年,彼得·米切尔提出,化学渗透作用是细胞的主要能量转换机制。这种机制现在在活细胞中无处不在,它为微生物和真核生物的线粒体中的能量转换提供动力,使其成为早期生命的可能候选。[159][160]线粒体可以产生三磷酸腺苷(ATP),是细胞的能量货币,可用于驱动化学合成等细胞过程。ATP合成机制涉及一个封闭的膜,其上镶嵌着ATP合酶,而释放强结合ATP所需的能量来自跨膜运动的质子。[161]在现代细胞中,跨膜的离子泵负责产生质子运动、维持电化学梯度。在第一个生物体中,这种梯度可能来自热液喷口的水流和周围的海水之间的化学成分差异,[150]在陆地上,陨石醌有利于发展跨脂质膜的化合能。[162]

RNA世界
[编辑]RNA世界学说认为,早期地球上有自我复制和催化的RNA,但没有DNA或蛋白质。[163]许多研究者一致认为,在现在占主导地位的DNA生命之前,一定存在过一个RNA世界。[15] 然而,基于RNA的生命可能也不是最早存在的生命体形式。[164][165]另一个模型与达尔文的“温暖的小池塘”相对,有干湿循环。[166]
RNA是翻译过程的核心。小RNA可以催化生命所需的大量化学反应,可以加速信息传递。[165][167]RNA在现代生物体中既能表达又能储存遗传信息;而且在接近早期地球的条件下,RNA很容易合成,这些与今天的条件大不相同。核酶的结构可视作“吸烟枪”,它有一个RNA的中心核心,在催化肽键形成的活性位点的18埃内没有氨基酸侧链。[12]:29[164][168]
RNA世界的概念由亚历山大·里奇在1962年提出,[169]沃特·吉尔伯特在1986年为其命了名。[165][170]最初,在解释胞嘧啶和尿嘧啶的非生物合成方面存在困难,[171]后来的研究显示了可能的合成途径,例如甲酰胺在各种陆地矿物存在的情况下,加温后会产生所有4种核糖核苷酸和其他生物分子。[125][126]

RNA复制酶既可以是储存遗传信息的编码,也可以是进一步进行RNA复制的催化剂,也就是说它可以实现自催化反应。杰克·绍斯塔克指出,某些催化性RNA可以将较短的RNA序列连接在一起,这就有了自我复制的可能。RNA复制系统包括两个相互催化的核糖酶,产物倍增时间约为1小时,且在实验条件下可进行自然选择。[172][173][164]如果早期地球上存在这样的条件,那么便有利于这种自催化组的繁衍,在自然选择下,便可以增加更多的功能。[174][175][176]RNA的自组装可能自发发生于热液喷口附近。[177][178][179]tRNA的初始形式可能已经组装成这样一个可复制分子。[180]
蛋白质合成的可能前体包括短肽辅因子的合成或RNA的自催化复制。原始核糖体很可能只由RNA组成,尽管有些作用后来改由蛋白质实现。这个话题剩下的主要问题,包括确定核糖体进化的选择力量,和确定遗传密码是如何产生的。[181]
尤金·库宁认为:“对于复制与翻译的起源,目前还没有令人信服的假说。这些关键过程共同构成了生命系统的核心和进化的前提。RNA世界假说可能为其解决提供了最好的机会,但迄今为止,还不能确切确定高效RNA复制酶或翻译系统的出现。”[182]
系统发育与LUCA
[编辑]自1977年卡尔·乌斯的研究开始,基因组学研究将所有现代生命的最后共同祖先(LUCA)置于细菌一支和古菌及真核生物组成的一支这样两支之间。其年代距今超过40亿年。[183][184]少数研究将LUCA置于细菌中,认为古菌和真核生物在进化上来自真细菌内;[185]汤玛斯·卡弗利尔-史密斯认为,LUCA可能属于表型多样的绿弯菌门。[186]
2016年,确认了可能存在于LUCA中的355个基因。一共测序了来自细菌和古菌的610万组原核生物基因,从286,514个蛋白簇中确定了355个可能存在于LUCA的蛋白簇。研究结果表明LUCA是嗜热厌氧生物,具有伍德-隆达尔代谢途径,可以固氮、固碳。其辅因子表明LUCA以来富含氢气、二氧化碳、铁和过渡金属的环境。其遗传物质可能是DNA,需要由4种核苷酸组成的遗传密码、信使RNA、转运RNA和核糖体以将遗传信息翻译为酶等蛋白质。LUCA可能生活在无氧的海底热泉附近,地球化学活动活跃。显然LUCA是一个复杂的生物体,且一定有前身,不是第一个生物。[30][187]LUCA的生理机能则有很大争议。[188][189][190]
-
LUCA系统与环境[30]
莱斯利·奥格尔认为,早期的翻译系统很容易受到差错成灾的影响。然而Geoffrey Hoffmann提出,这种系统在功能上是稳定的,足以对抗“奥格尔悖论”。[191][192][193]
适宜的地质环境
[编辑]深海热液喷口
[编辑]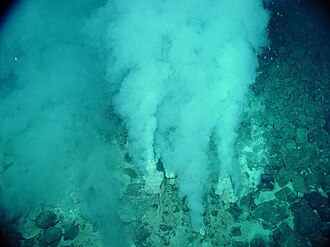
早期的微生物化石可能来自一个充满了甲烷、氨、二氧化碳和硫化氢的炎热世界,对目前的大多数生命来说都有毒。[194]对生命之树的分析将嗜热和超嗜热细菌及古菌置于最接近树根的地方,表明生命可能在炎热的环境中开始进化。[195]深海或碱性海底热泉理论认为,生命起源于海底热液喷口。[196][197]马丁和拉塞尔认为:“生命的进化始于渗漏点热液丘中的带纹理一硫化铁沉淀物,位于富含硫化物的热液,与富含亚铁的冥古宙深海之间,氧化还原、pH和温度均存在梯度变化。在渗漏点金属硫化物沉淀化石中,观察到了自然产生的立体隔层,这些无机隔层是独立生存的原核生物中发现的细胞壁、膜的前体。已知FeS和NiS有能力催化一氧化碳和甲基硫化物(热液的成分)的反应,生成乙酰-甲基硫化物,这表明,前生物合成过程主要发生在这些金属硫化物小室的内表面。”[198]
它们形成于富氢液体从海底涌出的地方,是超镁铁质橄榄石发生蛇纹岩化,以及与富含二氧化碳的海水维持pH界面的结果。海底热泉喷口可通过氧化还原反应源源不断地产生化学能,其中电子供体为分子氢,电子受体为二氧化碳;详见铁硫世界学说。它们都是放热反应。[196][c]
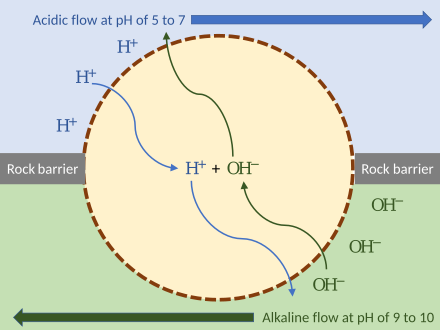
拉塞尔证明,碱性喷口创造了一个非生物源的质子动力化学渗透梯度,[198]是非生物源的理想选择。其上的微观小室“提供了集中有机分子的自然手段”,主要由铁、硫组成,如四方硫铁矿,这使得这些矿物小室产生了根特·瓦赫特绍泽设想的催化特性。[199]离子的这种跨膜运动取决于两个因素:
这两个梯度合起来可以表示为电化学梯度,可为非生物合成提供能量。质子动力可以视作是质子和跨膜电压梯度的差异(质子浓度与电动势差异)的组合所储存势能的量度。[150]
深海热液喷口内的矿物颗粒表面具有类似于酶的催化特性。若有外加电压驱动,或与H2或H2S反应,可以从水中溶解的二氧化碳生成简单的有机分子,如甲醇(CH3OH)、甲酸、乙酸和丙酮酸。[200][201]
马丁在2016年报告的研究支持生命产生于热液喷口的观点,[202][203]地壳中,由岩水相互作用,在不平衡热动力驱动下的自发化学作用支撑了生命的起源,[204][205]古菌和细菌源于依赖H2的自养生物,能量代谢最终产物为二氧化碳。[206]马丁认为,根据这一整局,LUCA“可能在很大程度上依赖喷口的地热能而生存”。[207]有人认为,那时深海热液喷口已没有孔隙,而被可促进生化反应的膜状隔间填满。[208][209]
温泉
[编辑]阿尔缅·穆尔基德亚尼安(Mulkidjanian)和合著者认为,海洋环境不能提供细胞中普遍存在的离子平衡和组成,也没有提供基本蛋白质和核酶所需的离子,特别是在高K+/Na+比率、Mn2+、Zn2+和磷酸盐浓度方面。他们认为,地球上唯一接近所需条件的环境,可能是堪察加半岛上的温泉。[210]在缺氧环境下,这些环境中的矿石表面会有适中的pH值(而在目前的富氧大气中的矿藏则没有),含有的光催化硫化物沉淀物可以吸收有害的紫外线,有干湿循环,可以将基质溶液浓缩到适合自发形成聚集物的浓度。[211][212]热液环境中的化学反应,以及从喷口到相邻的水池过程中暴露于紫外线下,都会促进生物大分子的形成。[213]这样推拟出来的前生物环境与热液喷口相似但不同,这有助于解释LUCA的特殊性。[210][162]
对具有久远历史的蛋白质进行的系统发育和地球化学分析表明,其细胞内液的离子成分与温泉里的相同。LUCA很可能通过摄取环境合成的有机物谋生。[210]实验表明,在多次干湿循环和紫外线辐射下,可以形成类似RNA的聚集物,并在凝结后被裹在囊泡中。由于离子溶质的浓度很高,盐水条件下不会发生这种情况。[214]温泉中生物大分子的一个可能来源是行星际尘埃粒子、地外弹射或大气/地球化学合成物运输。冥古宙的火山地块可能有大量温泉。[162]
黏土
[编辑]黏土假说是由格雷厄姆·凯恩斯-史密斯(Graham Cairns-Smith)于1982年提出的。[215][216]他推测,无机、可自我复制的硅酸盐晶体表面在与水溶液接触的过程中,逐渐产生了复杂有机分子。黏土矿物蒙脱石已被证明可以催化核苷酸单体在水溶液中聚集为RNA的反应,[217]并催化脂质形成膜。[218]1998年,海曼·哈特曼(Hyman Hartman)提出:“第一个生命体是可自我复制的富铁黏土,捕获二氧化碳并合成草酸和其他二羧酸。这种可复制的黏土系统及其代谢表型,随后进化到温泉中的富硫区域,于是获得了固氮的能力。最终,磷酸盐被纳入不断进化的系统中,使得核苷酸和磷脂的合成成为可能。”[219]
铁硫世界
[编辑]1980年代,根特·瓦赫特绍泽和卡尔·波普尔提出了铁硫世界学说,用以解释前生物化学途径的进化。它将今日的生物化学追溯到从气体中合成有机构件的原始反应。[220][221]瓦赫特绍泽系统有一个取之不尽的能量来源前提:铁硫化物,如黄铁矿。氧化这些金属硫化物所释放的能量可以支持有机分子的合成。这样的系统可能演变为自催化组,构成了自我复制、代谢活跃的实体,早于现代生命形式的出现。[16]在100°C的水环境中用硫化物进行实验,产生了少量二肽(0.4-12.4%)和少量三肽(0.10%)。但在同样的条件下,二肽分解得很快。[222]
有些模型专门试图推测原始的新陈代谢,RNA复制的顺序就要往后放。三羧酸循环的核心地位无论是在有氧生物的能量生产中,还是在复杂有机物的生物合成中吸收二氧化碳和氢离子方面都无可动摇,表明它是新陈代谢中最早出现的部分之一。[199]同时,地球化学家杰克·绍斯塔克和凯特·阿达玛拉(Kate Adamala)证明,只有在柠檬酸等弱阳离子螯合剂存在时,原始原生细胞才有可能进行非酶性RNA复制。这为柠檬酸在原始代谢中的核心作用提供了进一步的证据。[223] 拉塞尔认为“生命的目的是使二氧化碳氢化”(作为“代谢在先”而非“繁殖在先”的假说的一部分)。[224][225][16]物理学家杰里米·英格兰从一般的热力学视角出发,认为生命的产生不可避免。[226]这种想法来自奥巴林于1924年提出的可自我复制的囊泡。1980与1990年代,先后出现了瓦赫特绍泽的铁硫世界假说和克里斯蒂安·德·迪夫的硫酯模型。无需基因的代谢等更抽象的理论论证,包括弗里曼·戴森的数学模型,和斯图亚特·考夫曼在1980年代提出的自催化集团,后者忽视了能量在驱动细胞内生化反应中的作用。[227]

三羧酸循环这样的多步生化途径不会是在矿物表面自发形成的,之前一定有更简单的途径。[228]伍德-隆达尔代谢途径与金属硫化物表面的自组织相匹配。它的关键酶单元一氧化碳脱氢酶/乙酰辅酶A合成酶在反应中心含有混合的镍-铁-硫簇,可以催化乙酰辅酶A的合成。然而,在假设的热液喷口的前生物条件下,前生物的硫醇、硫酯化合物不太可能在热力学和动力学的视角下积累。[229]一种可能性是,半胱氨酸和高半胱氨酸可能与来自斯特雷克反应的腈类物质发生反应,形成富含硫醇的可催化多肽。[230]
锌世界
[编辑]阿尔缅的锌世界假说扩展了瓦赫特绍泽的黄铁矿假说。[231][232]锌世界假说认为,富含H2S的热液与寒冷的原始海洋(或达尔文所谓“温暖的小池塘”)里的水相互作用,使得金属硫化物颗粒沉淀。海洋热液系统存在地带性结构,反映在古代火成块状硫化物矿床中,它们的直径可达许多千米,多诞生于太古宙。最常见的是黄铁矿(FeS2)、黄铜矿(CuFeS2)和闪锌矿(ZnS),还有少量方铅矿(PbS)和硫锰矿(MnS)。ZnS与MnS具有储存辐射能量的独特能力,例如来自紫外线的辐射。自我复制分子起源时,原始大气压高到足以在地表附近析出(>100巴),紫外线也比现在强10到100倍;因此,以ZnS为中介的光合特性,为信息和代谢分子的合成,以及耐光核碱基的选择提供了合适的能量条件。[231][233]
锌世界假说可在第一批原生细胞内的离子构成找到证据。1926年,加拿大生物化学家阿奇巴尔德·马卡伦(Archibald Macallum)注意到血、淋巴等体液的成分与海水相似;[234]然而,所有细胞的无机成分都与现代海水的无机成分不同,这使得阿尔缅及同事结合地球化学分析和现代细胞无机离子需求的系统基因组学审查,重建了第一批细胞的“孵化室”。作者的结论是,无处不在的、基本的蛋白质和功能系统普遍显示出对K+、Zn2+、Mn2+和[PO
4]3−
的需求。地球化学构拟表明,这种离子组成不可能存在于海洋中,但契合内陆地热系统。在以二氧化碳为主的原始大气下,地温梯度场附近的水凝结物的化学成分与现代细胞的内部环境相似。因此,细胞前的进化可能发生在较浅的“达尔文池”中,池内有多孔硅酸盐矿物与金属硫化物,并富含K+、Zn2+和磷化合物。[235][236]
同手性
[编辑]
生物使用具有相同手性的分子。[238]:353–364氨基酸均为左手性,核苷酸和糖类均为右手性,几乎没有例外。手性分子可以从非生物途径获得,但在没有手性源或手性催化剂的情况下,它们两种形式会各占一半(外消旋)。已知的从外消旋底物产生非外消旋混合物的机制包括:不对称的物理规律,如电弱相互作用;不对称的环境,如圆偏振光、石英晶体或受地球自转影响显著的环境;外消旋合成过程中的统计涨落[237]和自发对称破缺。[239][240][241]一旦建立起来,手性就会被选择出来。[242]群体中的小偏向(对映体过剩)可以通过不对称自催化放大,例如硖合反应。[243]在不对称自催化中,催化剂是手性分子,意味着一个手性分子正在催化自身的复制。最初的对映体过剩可能由偏振光产生,之后更丰富的对映体便会开始与另一个对映体发生竞争。[244]同手性可能始于外太空,因为在默奇森陨石上,L丙氨酸的含量是其D型的两倍多,而L谷氨酸是其D型的三倍多。[245]:184[246]来自陨石的氨基酸显示出偏向左手的手性,而糖类则偏右手,这与在地球生物体内发现的一样,表明这些化合物可能起源自外太空。[247]
总体过程
[编辑]-
热液喷口中的原细胞可以通过将脂肪酸添加到细胞膜中、将其他有机物添加到细胞质中来生长。[250]
-
在热液喷口中的原细胞中,核苷酸可以聚集成随机的RNA链。任何具有轻微催化活性的RNA都会促进其原细胞的生长和复制,从而开始自然选择。[251]
-
无氧光合作用利用硫化氢取代稀缺的氢气。[253]
-
早期异养生物使用三羧酸循环呼吸;然后氧气光合作用使其完全独立于海底火山的能量。[253]
参见
[编辑]注释
[编辑]- ^ 有时称为生物创建 (Bernal, 1960, p. 30)
- ^ 反应是:
- FeS + H2S → FeS2 + 2H+ + 2e−
- FeS + H2S + CO2 → FeS2 + HCOOH
- ^ 反应为:
反应1:- 3Fe2SiO4 + 2H2O → 2Fe3O4 + 3SiO2 + 2H2
- 3Mg2SiO4 + SiO2 + 4H2O → 2Mg3Si2O5(OH)4
- 2Mg2SiO4 + 3H2O → Mg3Si2O5(OH)4 + Mg(OH)2
- 2 Ca2SiO4 + 4 H2O → 3 CaO · 2 SiO2 · 3 H2O + Ca(OH)2
参考文献
[编辑]- ^ 1.0 1.1 Walker, Sara I.; Packard, N.; Cody, G. D. Re-conceptualizing the origins of life. Philosophical Transactions of the Royal Society A. 2017-11-13, 375 (2109): 20160337. Bibcode:2017RSPTA.37560337W. PMC 5686397
. PMID 29133439. doi:10.1098/rsta.2016.0337.
- ^ Oparin, Aleksandr Ivanovich. The Origin of Life. Phoenix Edition Series. 由Morgulis, Sergius翻译 2. Mineola, New York: Courier Corporation. 19382003 [2018-06-16]. ISBN 978-0486495224. (原始内容存档于2014-09-20).
- ^ 3.0 3.1 3.2 3.3 Peretó, Juli. Controversies on the origin of life (PDF). International Microbiology. 2005, 8 (1): 23–31 [2015-06-01]. PMID 15906258. (原始内容 (PDF)存档于2015-08-24).
自20世纪20年代亚历山大·奥帕林作出历史性贡献以来,对生命起源之谜的智力挑战就在这样的假设基础上展开:生命在地球上的起源是可以推测、理解和模拟的物理化学过程;也就是说,既没有神迹,也没有自然发生。
- ^ 4.0 4.1 比较:Scharf, Caleb; et al. A Strategy for Origins of Life Research. Astrobiology. 2015-12-18, 15 (12): 1031–1042. Bibcode:2015AsBio..15.1031S. PMC 4683543
. PMID 26684503. doi:10.1089/ast.2015.1113.
我们所说的生命的起源(origins of life)是什么意思?[......]自20世纪初以来,“生命的起源”一词被用来指地球上从非生物系统向生物系统过渡期间发生的事件,即地球生物的起源(Oparin, 1924; Haldane, 1929)。该术语在很大程度上取代了早期的概念,如生物起源(Kamminga, 1980; Fry, 2000)。
- ^ 5.0 5.1 Oparin 1953
- ^ Warmflash, David; Warmflash, Benjamin. Did Life Come from Another World?. Scientific American. 2005-11, 293 (5): 64–71. Bibcode:2005SciAm.293e..64W. PMID 16318028. doi:10.1038/scientificamerican1105-64.
根据传统的假说,最早的生物是数十亿年前地球上的化学进化的结果,这个过程也叫做“非生物生成”。
- ^ Yarus 2010
- ^ Witzany, Guenther. Crucial steps to life: From chemical reactions to code using agents (PDF). BioSystems. 2016, 140: 49–57 [2023-01-19]. PMID 26723230. doi:10.1016/j.biosystems.2015.12.007. (原始内容存档 (PDF)于2021-10-07).
- ^ 9.0 9.1 9.2 Howell, Elizabeth. How Did Life Become Complex, And Could It Happen Beyond Earth?. Astrobiology Magazine. 2014-12-08 [2022-04-14]. 原始内容存档于2018-02-15.
- ^ 10.0 10.1 Tirard, Stephane. Abiogenesis – Definition. 2015-04-20: 1. ISBN 978-3-642-27833-4. doi:10.1007/978-3-642-27833-4_2-4.
托马斯-赫胥黎(1825-1895)在1870年发表的一篇重要文章中,提到“非生物生成”。他严格区分了自发生成(他不接受自发生成)和物质在没有生命影响时,从惰性到生物的进化的可能性……自19世纪末以来,进化型生物生成是指在原始地球进化的非生物背景下,物质从惰性到生命状态的复杂性和进化性的增加。
- ^ Levinson, Gene. Rethinking evolution: the revolution that's hiding in plain sight. World Scientific. 2020 [2023-01-20]. ISBN 978-1786347268. (原始内容存档于2022-05-21).
- ^ 12.0 12.1 Voet & Voet 2004
- ^ Dyson 1999
- ^ Ward, Peter; Kirschvink, Joe. A New History of Life: the radical discoveries about the origins and evolution of life on earth. Bloomsbury Press. 2015: 39–40. ISBN 978-1608199105.
- ^ 15.0 15.1 * Copley, Shelley D.; Smith, Eric; Morowitz, Harold J. The origin of the RNA world: Co-evolution of genes and metabolism (PDF). Bioorganic Chemistry. 2007-12, 35 (6): 430–443 [2015-06-08]. PMID 17897696. doi:10.1016/j.bioorg.2007.08.001. (原始内容存档 (PDF)于2013-09-05).
The proposal that life on Earth arose from an RNA world is widely accepted.
- Orgel, Leslie E. Some consequences of the RNA world hypothesis. Origins of Life and Evolution of Biospheres. 2003-04, 33 (2): 211–218. Bibcode:2003OLEB...33..211O. PMID 12967268. S2CID 32779859. doi:10.1023/A:1024616317965.
It now seems very likely that our familiar DNA/RNA/protein world was preceded by an RNA world...
- Robertson & Joyce 2012:“现在有强有力的证据表明,在基于DNA和蛋白质的生命之前,确实存在一个RNA世界。”
- Neveu, Kim & Benner 2013:“[RNA世界的存在]在今天的社区内得到了广泛的支持。”
- Orgel, Leslie E. Some consequences of the RNA world hypothesis. Origins of Life and Evolution of Biospheres. 2003-04, 33 (2): 211–218. Bibcode:2003OLEB...33..211O. PMID 12967268. S2CID 32779859. doi:10.1023/A:1024616317965.
- ^ 16.0 16.1 16.2 Keller, Markus A.; Turchyn, Alexandra V.; Ralser, Markus. Non-enzymatic glycolysis and pentose phosphate pathway-like reactions in a plausible Archean ocean. Molecular Systems Biology. 2014-03-25, 10 (725): 725. PMC 4023395
. PMID 24771084. doi:10.1002/msb.20145228.
- ^ 17.0 17.1 Graham, Robert W. Extraterrestrial Life in the Universe (PDF). NASA (NASA Technical Memorandum 102363) (Lewis Research Center, Cleveland, Ohio). February 1990 [2015-06-02]. (原始内容存档 (PDF)于2014-09-03).
- ^ 18.0 18.1 Altermann 2009
- ^ 19.0 19.1 Age of the Earth. United States Geological Survey. 2007-07-09 [2006-01-10]. (原始内容存档于2005-12-23).
- ^ Manhesa, Gérard; Allègre, Claude J.; Dupréa, Bernard; Hamelin, Bruno. Lead isotope study of basic-ultrabasic layered complexes: Speculations about the age of the earth and primitive mantle characteristics. Earth and Planetary Science Letters. 1980-05, 47 (3): 370–382. Bibcode:1980E&PSL..47..370M. doi:10.1016/0012-821X(80)90024-2.
- ^ 21.0 21.1 Schopf, J. William; Kudryavtsev, Anatoliy B.; Czaja, Andrew D.; Tripathi, Abhishek B. Evidence of Archean life: Stromatolites and microfossils. Precambrian Research. 2007-10-05, 158 (3–4): 141–155. Bibcode:2007PreR..158..141S. doi:10.1016/j.precamres.2007.04.009.
- ^ 22.0 22.1 Schopf, J. William. Fossil evidence of Archaean life. Philosophical Transactions of the Royal Society B. 2006-06-29, 361 (1470): 869–885. PMC 1578735
. PMID 16754604. doi:10.1098/rstb.2006.1834.
- ^ 23.0 23.1 Raven & Johnson 2002:68
- ^ 24.0 24.1 24.2 24.3 Dodd, Matthew S.; Papineau, Dominic; Grenne, Tor; et al. Evidence for early life in Earth's oldest hydrothermal vent precipitates. Nature. 2017-03-01, 543 (7643): 60–64 [2017-03-02]. Bibcode:2017Natur.543...60D. PMID 28252057. doi:10.1038/nature21377
. (原始内容存档于2017-09-08).
- ^ 25.0 25.1 25.2 NASA Astrobiology Strategy (PDF). NASA. 2015 [2017-09-24]. (原始内容 (PDF)存档于2016-12-22).
- ^ Trifonov, Edward N. Vocabulary of Definitions of Life Suggests a Definition. Journal of Biomolecular Structure and Dynamics. 2011-03-17, 29 (2): 259–266. PMID 21875147. S2CID 38476092. doi:10.1080/073911011010524992
.
- ^ Voytek, Mary A. About Life Detection. NASA. 2021-03-06 [2021-03-08]. (原始内容存档于2021-03-18).
- ^ 28.0 28.1 Witzany, Guenther. Crucial steps to life: From chemical reactions to code using agents (PDF). BioSystems. 2016, 140: 49–57 [2023-01-19]. PMID 26723230. S2CID 30962295. doi:10.1016/j.biosystems.2015.12.007. (原始内容存档 (PDF)于2021-10-07).
- ^ Oparin, Aleksandr Ivanovich. The Origin of Life. 由Morgulis, Sergius翻译 2. Mineola, New York: Courier. 2003 [1938] [2020-05-30]. ISBN 978-0486495224. (原始内容存档于2023-04-02).
- ^ 30.0 30.1 30.2 Weiss, M. C.; Sousa, F. L.; Mrnjavac, N.; Neukirchen, S.; Roettger, M.; Nelson-Sathi, S.; Martin, W.F. The physiology and habitat of the last universal common ancestor (PDF). Nature Microbiology. 2016, 1 (9): 16116 [2023-01-19]. PMID 27562259. S2CID 2997255. doi:10.1038/NMICROBIOL.2016.116. (原始内容存档 (PDF)于2023-01-29).
- ^ Warmflash, David; Warmflash, Benjamin. Did Life Come from Another World?. Scientific American. 2005-11, 293 (5): 64–71. Bibcode:2005SciAm.293e..64W. PMID 16318028. doi:10.1038/scientificamerican1105-64.
- ^ Yarus 2010,第47页
- ^ Ward, Peter; Kirschvink, Joe. A New History of Life: the radical discoveries about the origins and evolution of life on earth. Bloomsbury Press. 2015: 39–40. ISBN 978-1608199105.
- ^ Sheldon 2005
- ^ Lennox 2001
- ^ 36.0 36.1 Bernal 1967
- ^ Balme, D. M. Development of Biology in Aristotle and Theophrastus: Theory of Spontaneous Generation. Phronesis. 1962, 7 (1–2): 91–104. doi:10.1163/156852862X00052.
- ^ Ross 1652
- ^ Dobell 1960
- ^ Bondeson 1999
- ^ Levine, R.; Evers, C. The Slow Death of Spontaneous Generation (1668-1859). [2013-04-18]. (原始内容存档于2008-04-26).
- ^ Oparin 1953
- ^ Tyndall 1905,IV, XII (1876), XIII (1878)
- ^ Horneck, Gerda; Klaus, David M.; Mancinelli, Rocco L. Space Microbiology. Microbiology and Molecular Biology Reviews. 2010-03, 74 (1): 121–156. Bibcode:2010MMBR...74..121H. PMC 2832349
. PMID 20197502. doi:10.1128/MMBR.00016-09.
- ^ Wickramasinghe, Chandra. Bacterial morphologies supporting cometary panspermia: a reappraisal. International Journal of Astrobiology. 2011, 10 (1): 25–30. Bibcode:2011IJAsB..10...25W. CiteSeerX 10.1.1.368.4449
. S2CID 7262449. doi:10.1017/S1473550410000157.
- ^ Rampelotto, P. H. (2010). "Panspermia: A promising field of research". In: Astrobiology Science Conference. Abs 5224.
- ^ Chang, Kenneth. Visions of Life on Mars in Earth's Depths. The New York Times. 2016-09-12 [2016-09-12]. (原始内容存档于2016-09-12).
- ^ Letter no. 7471, Charles Darwin to Joseph Dalton Hooker, 1 February (1871). Darwin Correspondence Project. [2020-07-07]. (原始内容存档于2020-07-07).
- ^ Priscu, John C. Origin and Evolution of Life on a Frozen Earth. Arlington County, Virginia: National Science Foundation. [2014-03-01]. (原始内容存档于2013-12-18).
- ^ Marshall, Michael. Charles Darwin's hunch about early life was probably right. BBC News. 2020-11-11 [2020-11-11]. (原始内容存档于2020-11-11).
- ^ Bahadur, Krishna. Photochemical Formation of Self–sustaining Coacervates (PDF). Proceedings of the Indian National Science Academy. 1973, 39 (4): 455–467. PMID 1242552. doi:10.1016/S0044-4057(75)80076-1. (原始内容 (PDF)存档于2013-10-19).
- ^ Bahadur, Krishna. Photochemical Formation of Self-Sustaining Coacervates. Zentralblatt für Bakteriologie, Parasitenkunde, Infektionskrankheiten und Hygiene (Central Journal for Bacteriology, Parasitology, Infectious Diseases and Hygiene). 1975, 130 (3): 211–218 [2023-01-19]. OCLC 641018092. PMID 1242552. doi:10.1016/S0044-4057(75)80076-1. (原始内容存档于2022-12-13).
- ^ Bryson 2004
- ^ Bernal 1951
- ^ Martin, William F. On the origins of cells: a hypothesis for the evolutionary transitions from abiotic geochemistry to chemoautotrophic prokaryotes, and from prokaryotes to nucleated cells. Phil. Trans. R. Soc. Lond. A. 2003-01, 358 (1429): 59–83. PMC 1693102
. PMID 12594918. doi:10.1098/rstb.2002.1183.
- ^ Bernal, John Desmond. The Physical Basis of Life. Proceedings of the Physical Society, Section A. 1949-09, 62 (9): 537–558. Bibcode:1949PPSA...62..537B. S2CID 83754271. doi:10.1088/0370-1298/62/9/301.
- ^ Miller, Stanley L. A Production of Amino Acids Under Possible Primitive Earth Conditions. Science. 1953-05-15, 117 (3046): 528–529. Bibcode:1953Sci...117..528M. PMID 13056598. doi:10.1126/science.117.3046.528.
- ^ Parker, Eric T.; Cleaves, Henderson J.; Dworkin, Jason P.; et al. Primordial synthesis of amines and amino acids in a 1958 Miller H2S-rich spark discharge experiment. PNAS. 2011-04-05, 108 (14): 5526–5531. Bibcode:2011PNAS..108.5526P. PMC 3078417
. PMID 21422282. doi:10.1073/pnas.1019191108
.
- ^ Bernal 1967,第143页
- ^ 60.0 60.1 Cleaves, H. James; Chalmers, John H.; Lazcano, Antonio; et al. A Reassessment of Prebiotic Organic Synthesis in Neutral Planetary Atmospheres. Origins of Life and Evolution of Biospheres. 2008-04, 38 (2): 105–115. Bibcode:2008OLEB...38..105C. PMID 18204914. S2CID 7731172. doi:10.1007/s11084-007-9120-3.
- ^ Chyba, Christopher F. Rethinking Earth's Early Atmosphere. Science. 2005-05-13, 308 (5724): 962–963. PMID 15890865. S2CID 93303848. doi:10.1126/science.1113157.
- ^ Barton et al. 2007
- ^ Bada & Lazcano 2009
- ^ Bada, Jeffrey L.; Lazcano, Antonio. Prebiotic Soup – Revisiting the Miller Experiment (PDF). Science. 2003-05-02, 300 (5620): 745–746 [2015-06-13]. PMID 12730584. S2CID 93020326. doi:10.1126/science.1085145. (原始内容存档 (PDF)于2016-03-04).
- ^ Marigo, Paola; et al. Carbon star formation as seen through the non-monotonic initial–final mass relation. Nature Astronomy. 2020-06-06, 152 (11): 1102–1110 [2023-01-19]. Bibcode:2020NatAs...4.1102M. S2CID 220403402. arXiv:2007.04163
. doi:10.1038/s41550-020-1132-1. (原始内容存档于2023-02-16).
- ^ WMAP- Life in the Universe. [2023-01-19]. (原始内容存档于2023-01-29).
- ^ Formation of Solar Systems: Solar Nebular Theory. University of Massachusetts Amherst. [2019-09-27]. (原始内容存档于2019-09-27).
- ^ Dalrymple 2001
- ^ Fesenkov 1959
- ^ Kasting, James F. Earth's Early Atmosphere (PDF). Science. 1993-02-12, 259 (5097): 920–926 [2015-07-28]. Bibcode:1993Sci...259..920K. PMID 11536547. S2CID 21134564. doi:10.1126/science.11536547. (原始内容 (PDF)存档于2015-10-10).
- ^ Morse, John. Hadean Ocean Carbonate Geochemistry. Aquatic Geochemistry. 1998-09, 4 (3/4): 301–319. Bibcode:1998MinM...62.1027M. S2CID 129616933. doi:10.1023/A:1009632230875.
- ^ 72.0 72.1 72.2 72.3 72.4 72.5 72.6 72.7 Follmann, Hartmut; Brownson, Carol. Darwin's warm little pond revisited: from molecules to the origin of life. Naturwissenschaften. 2009-11, 96 (11): 1265–1292. Bibcode:2009NW.....96.1265F. PMID 19760276. S2CID 23259886. doi:10.1007/s00114-009-0602-1.
- ^ Morse, John W.; MacKenzie, Fred T. Hadean Ocean Carbonate Geochemistry. Aquatic Geochemistry. 1998, 4 (3–4): 301–319. Bibcode:1998MinM...62.1027M. S2CID 129616933. doi:10.1023/A:1009632230875.
- ^ Wilde, Simon A.; Valley, John W.; Peck, William H.; Graham, Colin M. Evidence from detrital zircons for the existence of continental crust and oceans on the Earth 4.4 Gyr ago (PDF). Nature. 2001-01-11, 409 (6817): 175–178 [2015-06-03]. Bibcode:2001Natur.409..175W. PMID 11196637. S2CID 4319774. doi:10.1038/35051550. (原始内容存档 (PDF)于2015-06-05).
- ^ Rosing, Minik T.; Bird, Dennis K.; Sleep, Norman H.; et al. The rise of continents – An essay on the geologic consequences of photosynthesis. Palaeogeography, Palaeoclimatology, Palaeoecology. 2006-03-22, 232 (2–4): 99–113 [2015-06-08]. Bibcode:2006PPP...232...99R. doi:10.1016/j.palaeo.2006.01.007. (原始内容存档 (PDF)于2015-07-14).
- ^ Gomes, Rodney; Levison, Hal F.; Tsiganis, Kleomenis; Morbidelli, Alessandro. Origin of the cataclysmic Late Heavy Bombardment period of the terrestrial planets. Nature. 2005-05-26, 435 (7041): 466–469. Bibcode:2005Natur.435..466G. PMID 15917802. doi:10.1038/nature03676
.
- ^ Sleep, Norman H.; Zahnle, Kevin J.; Kasting, James F.; et al. Annihilation of ecosystems by large asteroid impacts on early Earth. Nature. 1989-11-09, 342 (6246): 139–142. Bibcode:1989Natur.342..139S. PMID 11536616. S2CID 1137852. doi:10.1038/342139a0.
- ^ Chyba, Christopher; Sagan, Carl. Endogenous production, exogenous delivery and impact-shock synthesis of organic molecules: an inventory for the origins of life. Nature. 1992-01-09, 355 (6356): 125–132. Bibcode:1992Natur.355..125C. PMID 11538392. S2CID 4346044. doi:10.1038/355125a0.
- ^ Furukawa, Yoshihiro; Sekine, Toshimori; Oba, Masahiro; et al. Biomolecule formation by oceanic impacts on early Earth. Nature Geoscience. 2009-01, 2 (1): 62–66. Bibcode:2009NatGe...2...62F. doi:10.1038/NGEO383.
- ^ Maher, Kevin A.; Stevenson, David J. Impact frustration of the origin of life. Nature. 1988-02-18, 331 (6157): 612–614. Bibcode:1988Natur.331..612M. PMID 11536595. S2CID 4284492. doi:10.1038/331612a0.
- ^ Mann, Adam. Bashing holes in the tale of Earth's troubled youth. Nature. 2018-01-24, 553 (7689): 393–395. Bibcode:2018Natur.553..393M. doi:10.1038/d41586-018-01074-6
.
- ^ 82.0 82.1 Davies 1999
- ^ Bock & Goode 1996
- ^ Djokic, Tara; Van Kranendonk, Martin J.; Campbell, Kathleen A.; Walter, Malcolm R.; Ward, Colin R. Earliest signs of life on land preserved in ca. 3.5 Gao hot spring deposits. Nature Communications. 2017-05-09, 8: 15263. Bibcode:2017NatCo...815263D. PMC 5436104
. PMID 28486437. doi:10.1038/ncomms15263.
- ^ Schopf, J. William; Kitajima, Kouki; Spicuzza, Michael J.; Kudryavtsev, Anatolly B.; Valley, John W. SIMS analyses of the oldest known assemblage of microfossils document their taxon-correlated carbon isotope compositions. PNAS. 2017, 115 (1): 53–58. Bibcode:2018PNAS..115...53S. PMC 5776830
. PMID 29255053. doi:10.1073/pnas.1718063115
.
- ^ Tyrell, Kelly April. Oldest fossils ever found show life on Earth began before 3.5 billion years ago. University of Wisconsin-Madison. 2017-12-18 [2017-12-18]. (原始内容存档于2021-02-10).
- ^ Ohtomo, Yoko; Kakegawa, Takeshi; Ishida, Akizumi; et al. Evidence for biogenic graphite in early Archaean Isua metasedimentary rocks. Nature Geoscience. 2014-01, 7 (1): 25–28. Bibcode:2014NatGe...7...25O. doi:10.1038/ngeo2025.
- ^ Hassenkam, T.; Andersson, M. P.; Dalby, K. N.; Mackenzie, D.M.A.; Rosing, M.T. Elements of Eoarchean life trapped in mineral inclusions. Nature. 2017, 548 (7665): 78–81. Bibcode:2017Natur.548...78H. PMID 28738409. S2CID 205257931. doi:10.1038/nature23261.
- ^ Noffke, Nora; Christian, Daniel; Wacey, David; Hazen, Robert M. Microbially Induced Sedimentary Structures Recording an Ancient Ecosystem in the ca. 3.48 Gyo Dresser Formation, Pilbara, Western Australia. Astrobiology. 2013-11-16, 13 (12): 1103–1124. Bibcode:2013AsBio..13.1103N. PMC 3870916
. PMID 24205812. doi:10.1089/ast.2013.1030.
- ^ O'Donoghue, James. Oldest reliable fossils show early life was a beach. New Scientist. 2011-08-21, 211: 13. doi:10.1016/S0262-4079(11)62064-2. (原始内容存档于2015-06-30).
- ^ Wacey, David; Kilburn, Matt R.; Saunders, Martin; et al. Microfossils of sulphur-metabolizing cells in 3.4-billion-year-old rocks of Western Australia. Nature Geoscience. 2011-10, 4 (10): 698–702. Bibcode:2011NatGe...4..698W. doi:10.1038/ngeo1238.
- ^ Bell, Elizabeth A.; Boehnike, Patrick; Harrison, T. Mark; et al. Potentially biogenic carbon preserved in a 4.1 billion-year-old zircon. PNAS. 2015-10-19, 112 (47): 14518–14521. Bibcode:2015PNAS..11214518B. PMC 4664351
. PMID 26483481. doi:10.1073/pnas.1517557112
. Early edition, published online before print.
- ^ Baumgartner, Rafael; Van Kranendonk, Martin; Wacey, David; et al. Nano−porous pyrite and organic matter in 3.5-billion-year-old stromatolites record primordial life (PDF). Geology. 2019, 47 (11): 1039–1043 [2023-01-19]. Bibcode:2019Geo....47.1039B. S2CID 204258554. doi:10.1130/G46365.1. (原始内容存档 (PDF)于2020-12-05).
- ^ Djokic, Tara; Van Kranendonk, Martin; Cambell, Kathleen; Walter, Malcolm. Earliest signs of life on land preserved in ca. 3.5 Ga hot spring deposits. Nature Communications. 2017, 3.
- ^ Landau, Elizabeth. Building Blocks of Life's Building Blocks Come From Starlight. NASA. 2016-10-12 [2016-10-13]. (原始内容存档于2016-10-13).
- ^ 96.0 96.1 Ehrenfreund, Pascale; Cami, Jan. Cosmic carbon chemistry: from the interstellar medium to the early Earth.. Cold Spring Harbor Perspectives in Biology. 2010-12, 2 (12): a002097. PMC 2982172
. PMID 20554702. doi:10.1101/cshperspect.a002097.
- ^ Geballe, Thomas R.; Najarro, Francisco; Figer, Donald F.; et al. Infrared diffuse interstellar bands in the Galactic Centre region. Nature. 2011-11-10, 479 (7372): 200–202. Bibcode:2011Natur.479..200G. PMID 22048316. S2CID 17223339. arXiv:1111.0613
. doi:10.1038/nature10527.
- ^ Klyce 2001
- ^ 99.0 99.1 99.2 99.3 Hoover, Rachel. Need to Track Organic Nano-Particles Across the Universe? NASA's Got an App for That. Ames Research Center. NASA. 2014-02-21 [2015-06-22]. (原始内容存档于2015-09-06).
- ^ Goncharuk, Vladislav V.; Zui, O. V. Water and carbon dioxide as the main precursors of organic matter on Earth and in space. Journal of Water Chemistry and Technology. 2015-02, 37 (1): 2–3. S2CID 97965067. doi:10.3103/S1063455X15010026.
- ^ Abou Mrad, Ninette; Vinogradoff, Vassilissa; Duvernay, Fabrice; et al. Laboratory experimental simulations: Chemical evolution of the organic matter from interstellar and cometary ice analogs. Bulletin de la Société Royale des Sciences de Liège. 2015, 84: 21–32 [2015-04-06]. Bibcode:2015BSRSL..84...21A. (原始内容存档于2015-04-13).
- ^ Oba, Yasuhiro; et al. Identifying the wide diversity of extraterrestrial purine and pyrimidine nucleobases in carbonaceous meteorites. Nature Communications. 2022-04-26, 13 (2008): 2008. Bibcode:2022NatCo..13.2008O. PMC 9042847
. PMID 35473908. S2CID 248402205. doi:10.1038/s41467-022-29612-x.
- ^ 'Life chemical' detected in comet. BBC News (London). 2009-08-18 [2015-06-23]. (原始内容存档于2015-05-25).
- ^ Thompson, William Reid; Murray, B. G.; Khare, Bishun Narain; Sagan, Carl. Coloration and darkening of methane clathrate and other ices by charged particle irradiation: Applications to the outer solar system. Journal of Geophysical Research. 1987-12-30, 92 (A13): 14933–14947. Bibcode:1987JGR....9214933T. PMID 11542127. doi:10.1029/JA092iA13p14933.
- ^ Goldman, Nir; Tamblyn, Isaac. Prebiotic Chemistry within a Simple Impacting Icy Mixture. Journal of Physical Chemistry A. 2013-06-20, 117 (24): 5124–5131 [2023-01-19]. Bibcode:2013JPCA..117.5124G. PMID 23639050. S2CID 5144843. doi:10.1021/jp402976n. (原始内容存档于2018-07-21).
- ^ NASA Ames PAH IR Spectroscopic Database. NASA. [2015-06-17]. (原始内容存档于2015-06-29).
- ^ 107.0 107.1 107.2 Hudgins, Douglas M.; Bauschlicher, Charles W. Jr.; Allamandola, Louis J. Variations in the Peak Position of the 6.2 μm Interstellar Emission Feature: A Tracer of N in the Interstellar Polycyclic Aromatic Hydrocarbon Population. The Astrophysical Journal. 2005-10-10, 632 (1): 316–332. Bibcode:2005ApJ...632..316H. CiteSeerX 10.1.1.218.8786
. S2CID 7808613. doi:10.1086/432495.
- ^ 108.0 108.1 108.2 Des Marais, David J.; Allamandola, Louis J.; Sandford, Scott; et al. Cosmic Distribution of Chemical Complexity. Ames Research Center. Mountain View, California: NASA. 2009 [2015-06-24]. (原始内容存档于2014-02-27).
- ^ 109.0 109.1 Carey, Bjorn. Life's Building Blocks 'Abundant in Space'. Space.com (Watsonville, California: Imaginova). 2005-10-18 [2015-06-23]. (原始内容存档于2015-06-26).
- ^ García-Hernández, Domingo. A.; Manchado, Arturo; García-Lario, Pedro; et al. Formation of Fullerenes in H-Containing Planetary Nebulae. The Astrophysical Journal Letters. 2010-11-20, 724 (1): L39–L43. Bibcode:2010ApJ...724L..39G. S2CID 119121764. arXiv:1009.4357
. doi:10.1088/2041-8205/724/1/L39.
- ^ d'Ischia, Marco; Manini, Paola; Moracci, Marco; et al. Astrochemistry and Astrobiology: Materials Science in Wonderland?. International Journal of Molecular Sciences. 2019-08-21, 20 (17): 4079. PMC 6747172
. PMID 31438518. doi:10.3390/ijms20174079
.
- ^ Gudipati, Murthy S.; Yang, Rui. In-situ Probing of Radiation-induced Processing of Organics in Astrophysical Ice Analogs – Novel Laser Desorption Laser Ionization Time-of-flight Mass Spectroscopic Studies. The Astrophysical Journal Letters. 2012-09-01, 756 (1): L24. Bibcode:2012ApJ...756L..24G. S2CID 5541727. doi:10.1088/2041-8205/756/1/L24.
- ^ 113.0 113.1 Gallori, Enzo. Astrochemistry and the origin of genetic material. Rendiconti Lincei. 2011-06, 22 (2): 113–118. S2CID 96659714. doi:10.1007/s12210-011-0118-4.“在‘天体化学:空间和时间中的分子’研讨会上提交的论文(罗马,2010年11月4-5日),由国家文学院‘Guido Donegani’基金会赞助。”
- ^ Martins, Zita. Organic Chemistry of Carbonaceous Meteorites. Elements. 2011-02, 7 (1): 35–40. doi:10.2113/gselements.7.1.35.
- ^ Martins, Zita; Botta, Oliver; Fogel, Marilyn L.; et al. Extraterrestrial nucleobases in the Murchison meteorite. Earth and Planetary Science Letters. 2008-06-15, 270 (1–2): 130–136. Bibcode:2008E&PSL.270..130M. S2CID 14309508. arXiv:0806.2286
. doi:10.1016/j.epsl.2008.03.026.
- ^ Callahan, Michael P.; Smith, Karen E.; Cleaves, H. James, II; et al. Carbonaceous meteorites contain a wide range of extraterrestrial nucleobases. PNAS. 2011-08-23, 108 (34): 13995–13998. Bibcode:2011PNAS..10813995C. PMC 3161613
. PMID 21836052. doi:10.1073/pnas.1106493108
.
- ^ Steigerwald, John. NASA Researchers: DNA Building Blocks Can Be Made in Space. Goddard Space Flight Center. NASA. 2011-08-08 [2015-06-23]. (原始内容存档于2015-06-23).
- ^ Kwok, Sun; Zhang, Yong. Mixed aromatic–aliphatic organic nanoparticles as carriers of unidentified infrared emission features. Nature. 2011-11-03, 479 (7371): 80–83. Bibcode:2011Natur.479...80K. PMID 22031328. S2CID 4419859. doi:10.1038/nature10542.
- ^ Jørgensen, Jes K.; Favre, Cécile; Bisschop, Suzanne E.; et al. Detection of the simplest sugar, glycolaldehyde, in a solar-type protostar with ALMA (PDF). The Astrophysical Journal Letters. 2012, 757 (1): L4 [2015-06-23]. Bibcode:2012ApJ...757L...4J. S2CID 14205612. arXiv:1208.5498
. doi:10.1088/2041-8205/757/1/L4. (原始内容存档 (PDF)于2015-09-24).
- ^ Furukawa, Yoshihiro; Chikaraishi, Yoshito; Ohkouchi, Naohiko; et al. Extraterrestrial ribose and other sugars in primitive meteorites. PNAS. 2019-11-13, 116 (49): 24440–24445. Bibcode:2019PNAS..11624440F. PMC 6900709
. PMID 31740594. doi:10.1073/pnas.1907169116
.
- ^ Oró, Joan; Kimball, Aubrey P. Synthesis of purines under possible primitive earth conditions: II. Purine intermediates from hydrogen cyanide. Archives of Biochemistry and Biophysics. 1962-02, 96 (2): 293–313. PMID 14482339. doi:10.1016/0003-9861(62)90412-5.
- ^ Cleaves II, Henderson. The origin of the biologically coded amino acids. Journal of Theoretical Biology. 2010, 263 (4): 490–498. Bibcode:2010JThBi.263..490C. PMID 20034500. doi:10.1016/j.jtbi.2009.12.014.
- ^ Breslow, R. On the Mechanism of the Formose Reaction. Tetrahedron Letters. 1959, 1 (21): 22–26. doi:10.1016/S0040-4039(01)99487-0.
- ^ Oró, Joan. Mechanism of Synthesis of Adenine from Hydrogen Cyanide under Possible Primitive Earth Conditions. Nature. 1961-09-16, 191 (4794): 1193–1194. Bibcode:1961Natur.191.1193O. PMID 13731264. S2CID 4276712. doi:10.1038/1911193a0.
- ^ 125.0 125.1 Saladino, Raffaele; Crestini, Claudia; Pino, Samanta; et al. Formamide and the origin of life. (PDF). Physics of Life Reviews. 2012-03, 9 (1): 84–104 [2023-01-19]. Bibcode:2012PhLRv...9...84S. PMID 22196896. doi:10.1016/j.plrev.2011.12.002. hdl:2108/85168
. (原始内容存档 (PDF)于2023-01-27).
- ^ 126.0 126.1 Saladino, Raffaele; Botta, Giorgia; Pino, Samanta; et al. From the one-carbon amide formamide to RNA all the steps are prebiotically possible. Biochimie. 2012-07, 94 (7): 1451–1456. PMID 22738728. doi:10.1016/j.biochi.2012.02.018. hdl:11573/515604.
- ^ Marlaire, Ruth (编). NASA Ames Reproduces the Building Blocks of Life in Laboratory. Ames Research Center. NASA. 2015-03-03 [2015-03-05]. (原始内容存档于2015-03-05).
- ^ Ferus, Martin; Nesvorný, David; Šponer, Jiří; et al. High-energy chemistry of formamide: A unified mechanism of nucleobase formation. PNAS. 2015, 112 (3): 657–662. Bibcode:2015PNAS..112..657F. PMC 4311869
. PMID 25489115. doi:10.1073/pnas.1412072111
.
- ^ Basile, Brenda; Lazcano, Antonio; Oró, Joan. Prebiotic syntheses of purines and pyrimidines. Advances in Space Research. 1984, 4 (12): 125–131. Bibcode:1984AdSpR...4l.125B. PMID 11537766. doi:10.1016/0273-1177(84)90554-4.
- ^ Orgel, Leslie E. Prebiotic Adenine Revisited: Eutectics and Photochemistry. Origins of Life and Evolution of Biospheres. 2004-08, 34 (4): 361–369. Bibcode:2004OLEB...34..361O. PMID 15279171. S2CID 4998122. doi:10.1023/B:ORIG.0000029882.52156.c2.
- ^ Robertson, Michael P.; Miller, Stanley L. An efficient prebiotic synthesis of cytosine and uracil. Nature. 1995-06-29, 375 (6534): 772–774. Bibcode:1995Natur.375..772R. PMID 7596408. S2CID 4351012. doi:10.1038/375772a0.
- ^ Fox, Douglas. Did Life Evolve in Ice?. Discover. 2008-02 [2008-07-03]. (原始内容存档于2008-06-30).
- ^ Levy, Matthew; Miller, Stanley L.; Brinton, Karen; Bada, Jeffrey L. Prebiotic Synthesis of Adenine and Amino Acids Under Europa-like Conditions. Icarus. 2000-06, 145 (2): 609–613. Bibcode:2000Icar..145..609L. PMID 11543508. doi:10.1006/icar.2000.6365.
- ^ Menor-Salván, César; Ruiz-Bermejo, Marta; Guzmán, Marcelo I.; et al. Synthesis of Pyrimidines and Triazines in Ice: Implications for the Prebiotic Chemistry of Nucleobases. Chemistry: A European Journal. 2009-04-20, 15 (17): 4411–4418. PMID 19288488. doi:10.1002/chem.200802656.
- ^ Roy, Debjani; Najafian, Katayoun; von Ragué Schleyer, Paul. Chemical evolution: The mechanism of the formation of adenine under prebiotic conditions. PNAS. 2007-10-30, 104 (44): 17272–17277. Bibcode:2007PNAS..10417272R. PMC 2077245
. PMID 17951429. doi:10.1073/pnas.0708434104
.
- ^ Lancet, Doron. Systems Prebiology-Studies of the origin of Life. The Lancet Lab. Rehovot, Israel: Department of Molecular Genetics; Weizmann Institute of Science. 2014-12-30 [2015-06-26]. (原始内容存档于2015-06-26).
- ^ Segré, Daniel; Ben-Eli, Dafna; Deamer, David W.; Lancet, Doron. The Lipid World (PDF). Origins of Life and Evolution of Biospheres. 2001-02, 31 (1–2): 119–145. Bibcode:2001OLEB...31..119S. PMID 11296516. S2CID 10959497. doi:10.1023/A:1006746807104. (原始内容存档 (PDF)于2015-06-26).
- ^ 138.0 138.1 138.2 Chen, Irene A.; Walde, Peter. From Self-Assembled Vesicles to Protocells. Cold Spring Harbor Perspectives in Biology. 2010-07, 2 (7): a002170. PMC 2890201
. PMID 20519344. doi:10.1101/cshperspect.a002170.
- ^ Eigen, Manfred; Schuster, Peter. The Hypercycle. A Principle of Natural Self-Organization. Part A: Emergence of the Hypercycle (PDF). Naturwissenschaften. 1977-11, 64 (11): 541–65. Bibcode:1977NW.....64..541E. PMID 593400. S2CID 42131267. doi:10.1007/bf00450633. (原始内容 (PDF)存档于2016-03-03).
- Eigen, Manfred; Schuster, Peter. The Hypercycle. A Principle of Natural Self-Organization. Part B: The Abstract Hypercycle (PDF). Naturwissenschaften. 1978, 65 (1): 7–41. Bibcode:1978NW.....65....7E. S2CID 1812273. doi:10.1007/bf00420631. (原始内容 (PDF)存档于2016-03-03).
- Eigen, Manfred; Schuster, Peter. The Hypercycle. A Principle of Natural Self-Organization. Part C: The Realistic Hypercycle (PDF). Naturwissenschaften. 1978-07, 65 (7): 341–369. Bibcode:1978NW.....65..341E. S2CID 13825356. doi:10.1007/bf00439699. (原始内容 (PDF)存档于2016-06-16).
- ^ Markovitch, Omer; Lancet, Doron. Excess Mutual Catalysis Is Required for Effective Evolvability. Artificial Life. Summer 2012, 18 (3): 243–266. PMID 22662913. S2CID 5236043. doi:10.1162/artl_a_00064.
- ^ Tessera, Marc. Origin of Evolution versus Origin of Life: A Shift of Paradigm. International Journal of Molecular Sciences. 2011, 12 (6): 3445–3458. PMC 3131571
. PMID 21747687. doi:10.3390/ijms12063445
. Special Issue: "Origin of Life 2011"
- ^ Onsager, Lars. Reciprocal Relations in Irreversible Processes I and II. Physical Review. 1931, 37 (4): 405. Bibcode:1931PhRv...37..405O. doi:10.1103/PhysRev.37.405.
- ^ Onsager, Lars. Reciprocal Relations in Irreversible Processes I and II. Physical Review. 1931, (38): 2265. doi:10.1103/PhysRev.38.2265.
- ^ Prigogine, Ilya. An Introduction to the Thermodynamics of Irreversible Processes. New York: Wiley. 1967.
- ^ Exploring Life's Origins: Protocells. Exploring Life's Origins: A Virtual Exhibit. Arlington County, Virginia: National Science Foundation. [2014-03-18]. (原始内容存档于2014-02-28).
- ^ 146.0 146.1 146.2 Chen, Irene A. The Emergence of Cells During the Origin of Life. Science. 2006-12-08, 314 (5805): 1558–1559. PMID 17158315. doi:10.1126/science.1137541
.
- ^ Zimmer, Carl. What Came Before DNA?. Discover. 2004-06-26. (原始内容存档于2014-03-19).
- ^ Shapiro, Robert. A Simpler Origin for Life. Scientific American. 2007-06, 296 (6): 46–53. Bibcode:2007SciAm.296f..46S. PMID 17663224. doi:10.1038/scientificamerican0607-46. (原始内容存档于2015-06-14).
- ^ Chang 2007
- ^ 150.0 150.1 150.2 150.3 Lane, Nick. The Vital Question: Why Is Life The Way It Is?. Profile Books. 2015: 129–140. ISBN 978-1781250365.
- ^ Sharov, Alexei A.; Gordon, Richard. Life Before Earth. Habitability of the Universe Before Earth: Life Before Earth. Astrobiology Exploring Life on Earth and Beyond. Academic Press. 2018: 265–296 [2023-01-19]. ISBN 9780128119402. S2CID 117048600. doi:10.1016/B978-0-12-811940-2.00011-3. (原始内容存档于2022-04-30).
- ^ Ladyman, J.; Lambert, J.; Weisner, K. B. What is a Complex System?. European Journal of the Philosophy of Science. 2013, 3: 33–67. S2CID 18787276. doi:10.1007/s13194-012-0056-8.
- ^ Esposito, M.; Lindenberg, Katja; Van den Broeck, C. Entropy production as correlation between system and reservoir. New Journal of Physics. 2010, 12 (1): 013013. Bibcode:2010NJPh...12a3013E. S2CID 26657293. arXiv:0908.1125
. doi:10.1088/1367-2630/12/1/013013.
- ^ Bar-Nun, A.; Bar-Nun, N.; Bauer, S. H.; Sagan, Carl. Shock Synthesis of Amino Acids in Simulated Primitive Environments. Science. 1970-04-24, 168 (3930): 470–473. Bibcode:1970Sci...168..470B. PMID 5436082. S2CID 42467812. doi:10.1126/science.168.3930.470.
- ^ Anbar, Michael. Cavitation during Impact of Liquid Water on Water: Geochemical Implications. Science. 1968-09-27, 161 (3848): 1343–1344. Bibcode:1968Sci...161.1343A. PMID 17831346. doi:10.1126/science.161.3848.1343.
- ^ Dharmarathne, Leena; Grieser, Franz. Formation of Amino Acids on the Sonolysis of Aqueous Solutions Containing Acetic Acid, Methane, or Carbon Dioxide, in the Presence of Nitrogen Gas. The Journal of Physical Chemistry A. 2016-01-07, 120 (2): 191–199. Bibcode:2016JPCA..120..191D. PMID 26695890. doi:10.1021/acs.jpca.5b11858.
- ^ Patehebieke, Yeersen; Zhao, Ze-Run; Wang, Su; Xu, Hao-Xing; Chen, Qian-Qian; Wang, Xiao. Cavitation as a plausible driving force for the prebiotic formation of N9 purine nucleosides. Cell Reports Physical Science. 2021, 2 (3): 100375. Bibcode:2021CRPS....200375P. S2CID 233662126. doi:10.1016/j.xcrp.2021.100375.
- ^ Kalson, Natan-Haim; Furman, David; Zeiri, Yehuda. Cavitation-Induced Synthesis of Biogenic Molecules on Primordial Earth. ACS Central Science. 2017-09-11, 3 (9): 1041–1049. PMC 5620973
. PMID 28979946. S2CID 21409351. doi:10.1021/acscentsci.7b00325.
- ^ Muller, Anthonie W. J. Were the first organisms heat engines? A new model for biogenesis and the early evolution of biological energy conversion. Progress in Biophysics and Molecular Biology. 1995, 63 (2): 193–231. PMID 7542789. doi:10.1016/0079-6107(95)00004-7
.
- ^ Muller, Anthonie W. J.; Schulze-Makuch, Dirk. Thermal energy and the origin of life. Origins of Life and Evolution of Biospheres. 2006, 36 (2): 77–189. Bibcode:2006OLEB...36..177M. PMID 16642267. S2CID 22179552. doi:10.1007/s11084-005-9003-4.
- ^ Junge, Wolfgang; Nelson, Nathan. ATP Synthase. Annual Review of Biochemistry. 2015-06-02, 84 (1): 631–657. PMID 25839341. doi:10.1146/annurev-biochem-060614-034124.
- ^ 162.0 162.1 162.2 Damer, Bruce; Deamer, David. The Hot Spring Hypothesis for an Origin of Life. Astrobiology. 2020-04-01, 20 (4): 429–452. Bibcode:2020AsBio..20..429D. ISSN 1531-1074. PMC 7133448
. PMID 31841362. doi:10.1089/ast.2019.2045.
- ^ Benner, S. A.; Bell, E. A.; Biondi, E.; Brasser, R.; Carell, T.; Kim, H.-J.; Mojzsis, S. J.; Omran, A.; Pasek, M. A.; Trail, D. When Did Life Likely Emerge on Earth in an RNA-First Process?. ChemSystemsChem. 2020, 2 (2). doi:10.1002/syst.201900035
.
- ^ 164.0 164.1 164.2 Robertson, Michael P.; Joyce, Gerald F. The origins of the RNA world. Cold Spring Harbor Perspectives in Biology. 2012-05, 4 (5): a003608. PMC 3331698
. PMID 20739415. doi:10.1101/cshperspect.a003608.
- ^ 165.0 165.1 165.2 Cech, Thomas R. The RNA Worlds in Context. Cold Spring Harbor Perspectives in Biology. 2012-07, 4 (7): a006742. PMC 3385955
. PMID 21441585. doi:10.1101/cshperspect.a006742.
- ^ Pearce, Ben K. D.; Pudritz, Ralph E.; Semenov, Dmitry A.; Henning, Thomas K. Origin of the RNA world: The fate of nucleobases in warm little ponds. PNAS. 2017-10-24, 114 (43): 11327–11332. Bibcode:2017PNAS..11411327P. PMC 5664528
. PMID 28973920. arXiv:1710.00434
. doi:10.1073/pnas.1710339114
.
- ^ Yarus, Michael. Getting Past the RNA World: The Initial Darwinian Ancestor. Cold Spring Harbor Perspectives in Biology. 2011-04, 3 (4): a003590. PMC 3062219
. PMID 20719875. doi:10.1101/cshperspect.a003590.
- ^ Fox, George.E. Origin and evolution of the ribosome. Cold Spring Harbor Perspectives in Biology. 2010-06-09, 2 (9(a003483)): a003483. PMC 2926754
. PMID 20534711. doi:10.1101/cshperspect.a003483
.
- ^ Neveu, Marc; Kim, Hyo-Joong; Benner, Steven A. The 'Strong' RNA World Hypothesis: Fifty Years Old. Astrobiology. 2013-04-22, 13 (4): 391–403. Bibcode:2013AsBio..13..391N. PMID 23551238. doi:10.1089/ast.2012.0868.
- ^ Gilbert, Walter. Origin of life: The RNA world. Nature. 1986-02-20, 319 (6055): 618. Bibcode:1986Natur.319..618G. S2CID 8026658. doi:10.1038/319618a0
.
- ^ Orgel, Leslie E. The origin of life on Earth. Scientific American. 1994-10, 271 (4): 76–83. Bibcode:1994SciAm.271d..76O. PMID 7524147. doi:10.1038/scientificamerican1094-76.
- ^ Lincoln, Tracey A.; Joyce, Gerald F. Self-Sustained Replication of an RNA Enzyme. Science. 2009-02-27, 323 (5918): 1229–1232. Bibcode:2009Sci...323.1229L. PMC 2652413
. PMID 19131595. doi:10.1126/science.1167856.
- ^ Joyce, Gerald F. Evolution in an RNA world. Cold Spring Harbor Perspectives in Biology. 2009, 74 (Evolution: The Molecular Landscape): 17–23. PMC 2891321
. PMID 19667013. doi:10.1101/sqb.2009.74.004.
- ^ Szostak, Jack W. The Origins of Function in Biological Nucleic Acids, Proteins, and Membranes. Chevy Chase, Maryland: HHMI. 2015-02-05 [2015-06-16]. (原始内容存档于2015-07-14).
- ^ Bernstein, Harris; Byerly, Henry C.; Hopf, Frederick A.; et al. The Darwinian Dynamic. The Quarterly Review of Biology. 1983-06, 58 (2): 185–207. JSTOR 2828805. S2CID 83956410. doi:10.1086/413216.
- ^ Michod 1999
- ^ Palasek, Stan. Primordial RNA Replication and Applications in PCR Technology. 2013-05-23. arXiv:1305.5581v1
[q-bio.BM].
- ^ Vlassov, Alexander V.; Kazakov, Sergei A.; Johnston, Brian H.; et al. The RNA World on Ice: A New Scenario for the Emergence of RNA Information. Journal of Molecular Evolution. 2005-08, 61 (2): 264–273. Bibcode:2005JMolE..61..264V. PMID 16044244. S2CID 21096886. doi:10.1007/s00239-004-0362-7.
- ^ Nussinov, Mark D.; Otroshchenko, Vladimir A.; Santoli, Salvatore. The emergence of the non-cellular phase of life on the fine-grained clayish particles of the early Earth's regolith. BioSystems. 1997, 42 (2–3): 111–118. PMID 9184757. doi:10.1016/S0303-2647(96)01699-1.
- ^ Kühnlein, Alexandra; Lanzmich, Simon A.; Brun, Dieter. tRNA sequences can assemble into a replicator. ELife. 2021-03-02, 10. PMC 7924937
. PMID 33648631. doi:10.7554/eLife.63431
.
- ^ Noller, Harry F. Evolution of protein synthesis from an RNA world.. Cold Spring Harbor Perspectives in Biology. 2012-04, 4 (4): a003681. PMC 3312679
. PMID 20610545. doi:10.1101/cshperspect.a003681.
- ^ Koonin, Eugene V. The cosmological model of eternal inflation and the transition from chance to biological evolution in the history of life. Biology Direct. 2007-05-31, 2: 15. PMC 1892545
. PMID 17540027. doi:10.1186/1745-6150-2-15.
- ^ Boone, David R.; Castenholz, Richard W.; Garrity, George M. (编). The Archaea and the Deeply Branching and Phototrophic Bacteria. Bergey's Manual of Systematic Bacteriology. Springer. 2001. ISBN 978-0-387-21609-6. (原始内容存档于2014-12-25).[页码请求]
- ^ Woese, C. R.; Fox, G. E. Phylogenetic structure of the prokaryotic domain: the primary kingdoms.. PNAS. 1977, 7 (11): 5088–5090. Bibcode:1977PNAS...74.5088W. PMC 432104
. PMID 270744. doi:10.1073/pnas.74.11.5088
.
- ^ Valas, R. E.; Bourne, P. E. The origin of a derived superkingdom: how a gram-positive bacterium crossed the desert to become an archaeon. Biology Direct. 2011, 6: 16. PMC 3056875
. PMID 21356104. doi:10.1186/1745-6150-6-16.
- ^ Cavalier-Smith, Thomas. Rooting the tree of life by transition analyses. Biology Direct. 2006, 1: 19. PMC 1586193
. PMID 16834776. doi:10.1186/1745-6150-1-19.
- ^ Early life liked it hot. Nature. 2016, 535 (7613): 468. S2CID 49905802. doi:10.1038/535468b.
- ^ Gogarten, Johann Peter; Deamer, David. Is LUCA a thermophilic progenote?. Nature Microbiology. 2016-11-25, 1 (12): 16229 [2023-01-19]. ISSN 2058-5276. PMID 27886195. S2CID 205428194. doi:10.1038/nmicrobiol.2016.229. (原始内容存档于2020-04-03) (英语).
- ^ Catchpole, Ryan; Forterre, Patrick. The evolution of Reverse Gyrase suggests a non-hyperthermophilic Last Universal Common Ancestor. Molecular Biology and Evolution. 2019, 36 (12): 2737–2747 [2023-01-19]. PMC 6878951
. PMID 31504731. doi:10.1093/molbev/msz180. (原始内容存档于2023-01-27).
- ^ Berkemer, Sarah J.; McGlynn, Shawn E. A New Analysis of Archaea–Bacteria Domain Separation: Variable Phylogenetic Distance and the Tempo of Early Evolution. Molecular Biology and Evolution. 2020-08-08, 37 (8): 2332–2340 [2023-01-19]. PMC 7403611
. PMID 32316034. doi:10.1093/molbev/msaa089. (原始内容存档于2023-01-27).
- ^ Hoffmann, Geoffrey W. On the origin of the genetic code and the stability of the translation apparatus. Journal of Molecular Biology. 1974-06-25, 86 (2): 349–362. PMID 4414916. doi:10.1016/0022-2836(74)90024-2.
- ^ Orgel, Leslie E. The Maintenance of the Accuracy of Protein Synthesis and its Relevance to Ageing. PNAS. 1963-04, 49 (4): 517–521. Bibcode:1963PNAS...49..517O. PMC 299893
. PMID 13940312. doi:10.1073/pnas.49.4.517
.
- ^ Hoffmann, Geoffrey W. The Stochastic Theory of the Origin of the Genetic Code. Annual Review of Physical Chemistry. 1975-10, 26: 123–144. Bibcode:1975ARPC...26..123H. doi:10.1146/annurev.pc.26.100175.001011.
- ^ Brasier, M. D. Secret Chambers: The Inside Story of Cells and Complex Life. Oxford University Press. 2012: 298.
- ^ Ward, Peter & Kirschvink, Joe, op cit, p. 42
- ^ 196.0 196.1 Colín-García, M.; Heredia, A.; Cordero, G.; et al. Hydrothermal vents and prebiotic chemistry: a review. Boletín de la Sociedad Geológica Mexicana. 2016, 68 (3): 599–620. doi:10.18268/BSGM2016v68n3a13
. (原始内容存档于2017-08-18).
- ^ Schirber, Michael. Hydrothermal Vents Could Explain Chemical Precursors to Life. NASA Astrobiology: Life in the Universe. NASA. 2014-06-24 [2015-06-19]. (原始内容存档于2014-11-29).
- ^ 198.0 198.1 Martin, William; Russell, Michael J. On the origins of cells: a hypothesis for the evolutionary transitions from abiotic geochemistry to chemoautotrophic prokaryotes, and from prokaryotes to nucleated cells. Philosophical Transactions of the Royal Society B. 2003-01-29, 358 (1429): 59–83; discussion 83–85. PMC 1693102
. PMID 12594918. doi:10.1098/rstb.2002.1183.
- ^ 199.0 199.1 Lane 2009
- ^ Usher, Oli. Chemistry of seabed's hot vents could explain emergence of life (新闻稿). University College London. 2015-04-27 [2015-06-19]. (原始内容存档于2015-06-20).
- ^ Roldan, Alberto; Hollingsworth, Nathan; Roffey, Anna; et al. Bio-inspired CO2 conversion by iron sulfide catalysts under sustainable conditions. Chemical Communications. 2015-05, 51 (35): 7501–7504 [2015-06-19]. PMID 25835242. doi:10.1039/C5CC02078F
. (原始内容存档于2015-06-20).
- ^ Baross, J. A.; Hoffman, S. E. Submarine hydrothermal vents and associated gradient environments as sites for the origin and evolution of life. Origins of Life and Evolution of Biospheres. 1985, 15 (4): 327–345. Bibcode:1985OrLi...15..327B. S2CID 4613918. doi:10.1007/bf01808177.
- ^ Russell, M. J.; Hall, A. J. The emergence of life from iron monosulphide bubbles at a submarine hydrothermal redox and pH front. Journal of the Geological Society. 1997, 154 (3): 377–402. Bibcode:1997JGSoc.154..377R. PMID 11541234. S2CID 24792282. doi:10.1144/gsjgs.154.3.0377.
- ^ Amend, J. P.; LaRowe, D. E.; McCollom, T. M.; Shock, E. L. The energetics of organic synthesis inside and outside the cell. Philosophical Transactions of the Royal Society B. 2013, 368 (1622): 20120255. PMC 3685458
. PMID 23754809. doi:10.1098/rstb.2012.0255.
- ^ Shock, E. L.; Boyd, E. S. Geomicrobiology and microbial geochemistry:principles of geobiochemistry. Elements. 2015, 11: 389–394. doi:10.2113/gselements.11.6.395.
- ^ Martin, W.; Russell, M. J. On the origin of biochemistry at an alkaline hydrothermal vent. Philosophical Transactions of the Royal Society B. 2007, 362 (1486): 1887–1925. PMC 2442388
. PMID 17255002. doi:10.1098/rstb.2006.1881.
- ^ Weiss, Madeline C.; Sousa, Filipa L.; Mrnjavac, Natalia; Neukirchen, Sinje; Roettger, Mayo; Nelson-Sathi, Shijulal; Martin, William F. The physiology and habitat of the last universal common ancestor. Nature Microbiology. 2016-07-25, 1 (9): 16116. PMID 27562259. S2CID 2997255. doi:10.1038/nmicrobiol.2016.116.[失效链接]
- ^ Lane, Nick; Martin, William F. The Origin of Membrane Bioenergetics. Cell. 2012-12-21, 151 (7): 1406–1416 [2023-01-19]. ISSN 0092-8674. PMID 23260134. S2CID 15028935. doi:10.1016/j.cell.2012.11.050. (原始内容存档于2022-12-06) (英语).
- ^ Baaske, Philipp; Weinert, Franz M.; Duhr, Stefan; Lemke, Kono H.; Russell, Michael J.; Braun, Dieter. Extreme accumulation of nucleotides in simulated hydrothermal pore systems. Proceedings of the National Academy of Sciences. 2007-05-29, 104 (22): 9346–9351. ISSN 0027-8424. PMC 1890497
. PMID 17494767. doi:10.1073/pnas.0609592104
(英语).
- ^ 210.0 210.1 210.2 Mulkidjanian, Armen Y.; Bychkov, Andrew Yu.; Dibrova, Daria V.; Galperin, Michael Y.; Koonin, Eugene V. Origin of first cells at terrestrial, anoxic geothermal fields. Proceedings of the National Academy of Sciences. 2012-04-03, 109 (14): E821–30. Bibcode:2012PNAS..109E.821M. PMC 3325685
. PMID 22331915. doi:10.1073/pnas.1117774109
.
- ^ Chandru, Kuhan; Guttenberg, Nicholas; Giri, Chaitanya; et al. Simple prebiotic synthesis of high diversity dynamic combinatorial polyester libraries. Communications Chemistry. 2018-05-31, 1 (1). doi:10.1038/s42004-018-0031-1
.
- ^ Forsythe, Jay G.; Yu, Sheng-Sheng; Mamajanov, Irena; et al. Ester-Mediated Amide Bond Formation Driven by Wet–Dry Cycles: A Possible Path to Polypeptides on the Prebiotic Earth. Angewandte Chemie International Edition in English. 2015-08-17, 54 (34): 9871–9875. PMC 4678426
. PMID 26201989. doi:10.1002/anie.201503792.
- ^ Patel, Bhavesh H.; Percivalle, Claudia; Ritson, Dougal J.; Duffy, Colm. D.; Sutherland, John D. Common origins of RNA, protein and lipid precursors in a cyanosulfidic protometabolism. Nature Chemistry. 2015-03-16, 7 (4): 301–307. Bibcode:2015NatCh...7..301P. ISSN 1755-4330. PMC 4568310
. PMID 25803468. doi:10.1038/nchem.2202.
- ^ Deamer, David. Where Did Life Begin? Testing Ideas in Prebiotic Analogue Conditions. Life. 2021-02-10, 11 (2): 134. PMC 7916457
. PMID 33578711. doi:10.3390/life11020134
.
- ^ Cairns-Smith, Graham. Genetic Takeover and the Mineral Origins of Life. Cambridge: 剑桥大学出版社. 1982-09-02. ISBN 0-521-23312-7. OCLC 7875600.
- ^ Dawkins 1996,第148–161页
- ^ Huang, Wenhua; Ferris, James P. One-Step, Regioselective Synthesis of up to 50-mers of RNA Oligomers by Montmorillonite Catalysis. Journal of the American Chemical Society. 2006-07-12, 128 (27): 8914–8919. PMID 16819887. doi:10.1021/ja061782k.
- ^ Subramaniam, Anand Bala; Wan, Jiandi; Gopinath, Arvind; Stone, Howard A. Semi-permeable vesicles composed of natural clay (PDF). Soft Matter. 2011, 7 (6): 2600–2612 [2023-01-19]. Bibcode:2011SMat....7.2600S. S2CID 52253528. arXiv:1011.4711
. doi:10.1039/c0sm01354d. (原始内容存档 (PDF)于2022-12-03).
- ^ Hartman, Hyman. Photosynthesis and the Origin of Life. Origins of Life and Evolution of Biospheres. 1998, 28 (4–6): 515–521. Bibcode:1998OLEB...28..515H. PMID 11536891. S2CID 2464. doi:10.1023/A:1006548904157.
- ^ Yue-Ching Ho, Eugene. Evolutionary Epistemology and Sir Karl Popper's Latest Intellectual Interest: A First-Hand Report. Intellectus. July–September 1990, 15: 1–3. OCLC 26878740. (原始内容存档于2012-03-11).
- ^ Popper, Karl R. Pyrite and the origin of life. Nature. 1990-03-29, 344 (6265): 387. Bibcode:1990Natur.344..387P. S2CID 4322774. doi:10.1038/344387a0.
- ^ Huber, Claudia; Wächtershäuser, Günter. Peptides by Activation of Amino Acids with CO on (Ni,Fe)S Surfaces: Implications for the Origin of Life. Science. 1998-07-31, 281 (5377): 670–672. Bibcode:1998Sci...281..670H. PMID 9685253. doi:10.1126/science.281.5377.670.
- ^ Adamala, Katarzyna; Szostak, Jack W. Nonenzymatic Template-Directed RNA Synthesis Inside Model Protocells. Science. 2013-11-29, 342 (6162): 1098–1100. Bibcode:2013Sci...342.1098A. PMC 4104020
. PMID 24288333. doi:10.1126/science.1241888.
- ^ Musser, George. How Life Arose on Earth, and How a Singularity Might Bring It Down. Observations (Blog). 2011-09-23 [2015-06-17]. (原始内容存档于2015-06-17).
- ^ Carroll, Sean. Free Energy and the Meaning of Life. Cosmic Variance (Blog). Discover. 2010-03-10 [2015-06-17]. (原始内容存档于2015-07-14).
- ^ England, Jeremy L. Statistical physics of self-replication (PDF). Journal of Chemical Physics. 2013-09-28, 139 (12): 121923. Bibcode:2013JChPh.139l1923E. PMID 24089735. S2CID 478964. arXiv:1209.1179
. doi:10.1063/1.4818538. hdl:1721.1/90392. (原始内容存档 (PDF)于2015-06-04).
- ^ Fox, Ronald F. Review of Stuart Kauffman, The Origins of Order: Self-Organization and Selection in Evolution. Biophysical Journal. 1993-12, 65 (6): 2698–2699. Bibcode:1993BpJ....65.2698F. PMC 1226010
. doi:10.1016/s0006-3495(93)81321-3.
- ^ Orgel, Leslie E. Self-organizing biochemical cycles. PNAS. 2000-11-07, 97 (23): 12503–12507. Bibcode:2000PNAS...9712503O. PMC 18793
. PMID 11058157. doi:10.1073/pnas.220406697
.
- ^ Chandru, Kuhan; Gilbert, Alexis; Butch, Christopher; Aono, Masashi; Cleaves, Henderson James II. The Abiotic Chemistry of Thiolated Acetate Derivatives and the Origin of Life. Scientific Reports. 2016-07-21, 6 (29883): 29883. Bibcode:2016NatSR...629883C. PMC 4956751
. PMID 27443234. doi:10.1038/srep29883.
- ^ Vallee, Yannick; Shalayel, Ibrahim; Ly, Kieu-Dung; Rao, K. V. Raghavendra; Paëpe, Gael De; Märker, Katharina; Milet, Anne. At the very beginning of life on Earth: the thiol-rich peptide (TRP) world hypothesis. The International Journal of Developmental Biology. 2017-11-08, 61 (8–9): 471–478 [2023-01-19]. PMID 29139533. doi:10.1387/ijdb.170028yv
. (原始内容存档于2023-01-27).
- ^ 231.0 231.1 Mulkidjanian, Armen Y. On the origin of life in the zinc world: 1. Photosynthesizing, porous edifices built of hydrothermally precipitated zinc sulfide as cradles of life on Earth. Biology Direct. 2009-08-24, 4: 26. PMC 3152778
. PMID 19703272. doi:10.1186/1745-6150-4-26.
- ^ Wächtershäuser, Günter. Before Enzymes and Templates: Theory of Surface Metabolism. Microbiological Reviews. 1988-12, 52 (4): 452–484. PMC 373159
. PMID 3070320. doi:10.1128/MMBR.52.4.452-484.1988.
- ^ Mulkidjanian, Armen Y.; Galperin, Michael Y. On the origin of life in the zinc world. 2. Validation of the hypothesis on the photosynthesizing zinc sulfide edifices as cradles of life on Earth. Biology Direct. 2009-08-24, 4: 27. PMC 2749021
. PMID 19703275. doi:10.1186/1745-6150-4-27.
- ^ Macallum, A. B. The Paleochemistry of the body fluids and tissues. Physiological Reviews. 1926-04-01, 6 (2): 316–357. doi:10.1152/physrev.1926.6.2.316.
- ^ Mulkidjanian, Armen Y.; Bychkov, Andrew Yu.; Dibrova, Daria V.; et al. Origin of first cells at terrestrial, anoxic geothermal fields. PNAS. 2012-04-03, 109 (14): E821–E830. Bibcode:2012PNAS..109E.821M. PMC 3325685
. PMID 22331915. doi:10.1073/pnas.1117774109
.
- ^ See also Lankenau 2011,第225–286页, interconnecting the "Two RNA worlds" concept, and Davidovich, Chen; Belousoff, Matthew; Bashan, Anat; Yonath, Ada. The evolving ribosome: from non-coded peptide bond formation to sophisticated translation machinery. Research in Microbiology. 2009-09, 160 (7): 487–492. PMID 19619641. doi:10.1016/j.resmic.2009.07.004.
- ^ 237.0 237.1 Plasson, Raphaël; Kondepudi, Dilip K.; Bersini, Hugues; et al. Emergence of homochirality in far-from-equilibrium systems: Mechanisms and role in prebiotic chemistry. Chirality. 2007-08, 19 (8): 589–600. PMID 17559107. doi:10.1002/chir.20440. "Special Issue: Proceedings from the Eighteenth International Symposium on Chirality (ISCD-18), Busan, Korea, 2006"
- ^ Chaichian, Rojas & Tureanu 2014
- ^ Jafarpour, Farshid; Biancalani, Tommaso; Goldenfeld, Nigel. Noise-induced symmetry breaking far from equilibrium and the emergence of biological homochirality (PDF). Physical Review E. 2017, 95 (3): 032407 [2023-01-19]. Bibcode:2017PhRvE..95c2407J. PMID 28415353. doi:10.1103/PhysRevE.95.032407
. (原始内容存档 (PDF)于2023-04-02).
- ^ Jafarpour, Farshid; Biancalani, Tommaso; Goldenfeld, Nigel. Noise-induced mechanism for biological homochirality of early life self-replicators. Physical Review Letters. 2015, 115 (15): 158101. Bibcode:2015PhRvL.115o8101J. PMID 26550754. S2CID 9775791. arXiv:1507.00044
. doi:10.1103/PhysRevLett.115.158101.
- ^ Frank, F.C. On spontaneous asymmetric synthesis. Biochimica et Biophysica Acta. 1953, 11 (4): 459–463. PMID 13105666. doi:10.1016/0006-3002(53)90082-1.
- ^ Clark, Stuart. Polarized Starlight and the Handedness of Life. American Scientist. July–August 1999, 87 (4): 336. Bibcode:1999AmSci..87..336C. doi:10.1511/1999.4.336.
- ^ Shibata, Takanori; Morioka, Hiroshi; Hayase, Tadakatsu; et al. Highly Enantioselective Catalytic Asymmetric Automultiplication of Chiral Pyrimidyl Alcohol. Journal of the American Chemical Society. 1996-01-17, 118 (2): 471–472. doi:10.1021/ja953066g.
- ^ Soai, Kenso; Sato, Itaru; Shibata, Takanori. Asymmetric autocatalysis and the origin of chiral homogeneity in organic compounds. The Chemical Record. 2001, 1 (4): 321–332. PMID 11893072. doi:10.1002/tcr.1017.
- ^ Hazen 2005
- ^ Meierhenrich, Uwe. Amino acids and the asymmetry of life caught in the act of formation. Berlin: Springer. 2008: 76–79. ISBN 978-3540768869.
- ^ Mullen, Leslie. Building Life from Star-Stuff. Astrobiology Magazine. 2005-09-05. (原始内容存档于2015-07-14).
- ^ Lane 2023,第121–123页.
- ^ Lane 2023,第147–148页.
- ^ Lane 2023,第147–148, 152–153页.
- ^ Lane 2023,第148页.
- ^ Lane 2023,第148–150页.
- ^ 253.0 253.1 253.2 Lane 2023,第166–167页.
文献
[编辑]- Altermann, Wladyslaw. Introduction: A Roadmap to Fata Morgana?. Seckbach, Joseph; Walsh, Maud (编). From Fossils to Astrobiology: Records of Life on Earth and the Search for Extraterrestrial Biosignatures. Cellular Origin, Life in Extreme Habitats and Astrobiology 12. Dordrecht, the Netherlands; London: Springer. 2009. ISBN 978-1-4020-8836-0.
- Bada, Jeffrey L.; Lazcano, Antonio. The Origin of Life. Ruse, Michael; Travis, Joseph (编). Evolution: The First Four Billion Years. Foreword by EO Wilson. Cambridge: Belknap Press of Harvard University Press. 2009. ISBN 978-0-674-03175-3. OCLC 225874308.
- Barton, Nicholas H.; Briggs, Derek E.G.; Eisen, Jonathan A.; et al. Evolution. Cold Spring Harbor, New York: Cold Spring Harbor Laboratory Press. 2007. ISBN 978-0-87969-684-9. OCLC 86090399.
- Bastian, H. Charlton. The Modes of Origin of Lowest Organisms. London; New York: Macmillan and Company. 1871 [2015-06-06]. OCLC 42959303.
- Bernal, J. D. The Physical Basis of Life. London: Routledge & Kegan Paul. 1951.
- Bernal, J. D. The Problem of Stages in Biopoesis
. Florkin, M. (编). Aspects of the Origin of Life. International Series of Monographs on Pure and Applied Biology. Oxford, UK; New York: Pergamon Press. 1960. ISBN 978-1-4831-3587-8.
- Bernal, J. D. The Origin of Life
. The Weidenfeld and Nicolson Natural History. Translation of Oparin by Ann Synge. London: Weidenfeld & Nicolson. 1967.
- Bock, Gregory R.; Goode, Jamie A. (编). Evolution of Hydrothermal Ecosystems on Earth (and Mars?). Ciba Foundation Symposium 202. Chichester, UK; New York: John Wiley & Sons. 1996. ISBN 978-0-471-96509-1.
- Bondeson, Jan. The Feejee Mermaid and Other Essays in Natural and Unnatural History. Ithaca, NY: 康奈尔大学出版社. 1999. ISBN 978-0-8014-3609-3.
- Bryson, Bill. A Short History of Nearly Everything. London: Black Swan. 2004. ISBN 978-0-552-99704-1. OCLC 55589795.
- Calvin, Melvin. Chemical Evolution: Molecular Evolution Towards the Origin of Living Systems on the Earth and Elsewhere
. Oxford, UK: 克拉伦登出版社. 1969. ISBN 978-0-19-855342-7. OCLC 25220.
- Chaichian, Masud; Rojas, Hugo Perez; Tureanu, Anca. Physics and Life. Basic Concepts in Physics: From the Cosmos to Quarks. Undergraduate Lecture Notes in Physics. Berlin; Heidelberg: Springer Berlin Heidelberg. 2014. ISBN 978-3-642-19597-6. OCLC 900189038. S2CID 115247432. doi:10.1007/978-3-642-19598-3_12.
- Chang, Thomas Ming Swi. Artificial Cells: Biotechnology, Nanomedicine, Regenerative Medicine, Blood Substitutes, Bioencapsulation, and Cell/Stem Cell Therapy. Regenerative Medicine, Artificial Cells and Nanomedicine 1. Hackensack, New Jersey: World Scientific. 2007. ISBN 978-981-270-576-1. OCLC 173522612.
- Dalrymple, G. Brent. The age of the Earth in the twentieth century: a problem (mostly) solved. Lewis, C.L.E.; Knell, S.J. (编). The Age of the Earth: from 4004 BC to AD 2002. Geological Society Special Publication 190. London: Geological Society of London. 2001: 205–221. Bibcode:2001GSLSP.190..205D. ISBN 978-1-86239-093-5. OCLC 48570033. S2CID 130092094. doi:10.1144/gsl.sp.2001.190.01.14.
- Darwin, Charles. Darwin, Francis , 编. The Life and Letters of Charles Darwin, Including an Autobiographical Chapter 3 3rd. London: John Murray. 1887. OCLC 834491774.
- Davies, Paul. The Fifth Miracle: The Search for the Origin of Life. London: Penguin Books. 1999. ISBN 978-0-14-028226-9.
- Dawkins, Richard. The Blind Watchmaker Reissue with a new introduction. New York: W.W. Norton & Company. 1996. ISBN 978-0-393-31570-7. OCLC 35648431.
- Dobell, Clifford. Antony van Leeuwenhoek and His 'Little Animals'
. New York: Dover Publications. 1960.
- Dyson, Freeman. Origins of Life Revised. Cambridge, UK; New York: 剑桥大学出版社. 1999. ISBN 978-0-521-62668-2.
- Fesenkov, V. G. Some Considerations about the Primaeval State of the Earth. Oparin, A.I.; et al (编). The Origin of Life on the Earth. I.U.B. Symposium Series 1. Edited for the IUBMB by Frank Clark and R.L.M. Synge English-French-German. London; New York: Pergamon Press. 1959. ISBN 978-1-4832-2240-0. International Symposium on the Origin of Life on the Earth (held at Moscow, 19–24 August 1957)
- Hazen, Robert M. Genesis: The Scientific Quest for Life's Origin
. Washington, DC: Joseph Henry Press. 2005. ISBN 978-0-309-09432-0. OCLC 60321860.
- Huxley, Thomas Henry. VIII Biogenesis and Abiogenesis [1870]. Discourses, Biological and Geological. Collected Essays VIII Reprint. New York: Greenwood Press. 1968 [1897] [2023-01-19]. OCLC 476737627. (原始内容存档于2015-05-07).
- Klyce, Brig. Kingsley, Stuart A.; Bhathal, Ragbir , 编. Panspermia Asks New Questions. The Search for Extraterrestrial Intelligence (SETI) in the Optical Spectrum III 4273. Bellingham, WA: SPIE. 2001-01-22 [2015-06-09]. ISBN 0-8194-3951-7. doi:10.1117/12.435366. (原始内容存档于2013-09-03). Proceedings of the SPIE held at San Jose, California, 22–24 January 2001
- Lane, Nick. Life Ascending: The 10 Great Inventions of Evolution
1st American. New York: W.W. Norton & Company. 2009. ISBN 978-0-393-06596-1. OCLC 286488326.
- Lankenau, Dirk-Henner. Two RNA Worlds: Toward the Origin of Replication, Genes, Recombination and Repair. Egel, Richard; Lankenau, Dirk-Henner; Mulkidjanian, Armen Y. (编). Origins of Life: The Primal Self-Organization. Heidelberg: Springer. 2011. ISBN 978-3-642-21624-4. OCLC 733245537. doi:10.1007/978-3-642-21625-1.
- Lennox, James G. Aristotle's Philosophy of Biology: Studies in the Origins of Life Science. Cambridge Studies in Philosophy and Biology. Cambridge, UK; New York: Cambridge University Press. 2001. ISBN 978-0-521-65976-5.
- Michod, Richard E. Darwinian Dynamics: Evolutionary Transitions in Fitness and Individuality. Princeton, NJ: 普林斯顿大学出版社. 1999. ISBN 978-0-691-02699-2. OCLC 38948118.
- Oparin, A.I. The Origin of Life. Translation and new introduction by Sergius Morgulis 2nd. Mineola, NY: Dover Publications. 1953. ISBN 978-0-486-49522-4.
- Raven, Peter H.; Johnson, George B. Biology
6th. Boston: McGraw-Hill. 2002. ISBN 978-0-07-112261-0. OCLC 45806501.
- Ross, Alexander. Arcana Microcosmi II. London. 1652 [2023-01-19]. OCLC 614453394. (原始内容存档于2024-02-24).
- Sheldon, Robert B. Hoover, Richard B.; Levin, Gilbert V.; Rozanov, Alexei Y.; Gladstone, G. Randall , 编. Astrobiology and Planetary Missions. Astrobiology and Planetary Missions 5906. Bellingham, WA: SPIE: 59061I. 2005-09-22. ISBN 978-0-8194-5911-4. doi:10.1117/12.663480. (原始内容存档于2023-01-19) 使用
|archiveurl=
需要含有|url=
(帮助). Proceedings of the SPIE held at San Diego, California, 31 July–2 August 2005 - Tyndall, John. Fragments of Science 2 6th. New York: P.F. Collier & Sons. 1905. OCLC 726998155.
- Voet, Donald; Voet, Judith G. Biochemistry 1 3rd. New York: John Wiley & Sons. 2004. ISBN 978-0-471-19350-0.
- Yarus, Michael. Life from an RNA World: The Ancestor Within. Cambridge, Massachusetts: 哈佛大学出版社. 2010: 287. ISBN 978-0-674-05075-4.
- Lane, Nick. Transformer: The Deep Chemistry of Life and Death. Profile Books. 2023. ISBN 978-1-7881-6055-1.
外部链接
[编辑]关于生命起源 的图书馆资源 |