用户:Koala0090/昆虫形态学

昆虫形态学是研究及描述昆虫身体形态的学科。由于昆虫与其他节肢动物在演化上相关,两者的术语会相当类似。昆虫具有三个能与其他节肢动物鉴别的主要特征:昆虫的身体分成三个部分(头节、胸节和腹节);拥有三对足;口器位于头壳(head capsule)之外。口器的位置将昆虫和他们同是六足亚门但非昆虫的近亲区分开,包含原尾目、双尾目和弹尾目等。
昆虫各类群间的解剖学差异可能极大。有身长 0.3 mm 的缨小蜂(fairflies),也有展翅宽可达 30 cm 的强喙夜蛾(great owlet moth)[1]:7;有些物种有很多眼睛,有些却一个都没有;有些物种具有发达的翅膀,有些却连翅膀都没有;有些物种则演化出擅长奔跑、跳跃、游泳,甚至挖掘的足;这些特化构造,使昆虫几乎占据了地球上除深海和南极洲之外的所有的生态栖位。本篇将介绍昆虫的基本结构,以及身体各部分的一些重要变异,并介绍一些形容昆虫解剖构造的术语及其定义。
解剖学概述
[编辑]昆虫就如同其他节肢动物一样,仅有外骨骼而没有内骨骼。外骨骼是一种通常由几丁质组成的坚硬外壳,可以保护并支撑身体。昆虫身体可区分为三个体段:头、胸节和腹节[2]。头部专门感知外界和摄取食物;胸节具有足和翅(有些昆虫无翅)的基部,专门进行运动;腹节则负责消化、呼吸、排泄,以及繁殖[1]:22–48。虽然所有昆虫身体三体段的功能相似,基本结构上却有巨大的差别,不同群体的翅、腿、触角和口器能明显的被做出区别[3]。
外部型态
[编辑]外骨骼
[编辑]昆虫的外骨骼又称表皮层(cuticle),表皮层又可分为两层;外层称作上表皮(epicuticle),薄、覆盖蜡、防水且不含几丁质。在其底下为由几丁质组成的原表皮(procuticle),且厚于上表皮。原表皮可分为2层,外层是外表皮(exocuticle),内层是内表皮(endocuticle)。内表皮是由无数层纤维性几丁质和蛋白质所构成,质地坚韧且具弹性,如同三明治般层层交叠而成;外表皮骨质化,质地较为坚硬[1]:22–24。许多身体较软昆虫的外表皮非常薄,特别是幼虫阶段(如毛虫)。就化学性质而言,几丁质是N-乙酰葡糖胺的长链聚合物,一种葡萄糖的衍生物。在未经化学修饰前,几丁质易弯曲、半透明、具弹性且坚韧。但就节肢动物而言,几丁质往往以经过修饰的形式出现,嵌入在硬化的蛋白质网格中,形成大部分的外骨骼。纯几丁质材质类似皮革,但当外面裹着碳酸钙时,他的硬度会大幅提升[4]。修饰与未修饰的差别可以透过比较毛虫(未修饰)和甲虫(已修饰)的体壁的得知。
外表皮及其底部的内基底膜,源自于胚胎期间的单层柱状或立方上皮细胞。内表皮则储备了昆虫大部分的构成原料。表皮提供肌肉支持躯体和保护昆虫。然而,因为表皮无法增长,外部骨化的部分会周期性的脱落,也就是蜕皮(ecdysis)。当快要蜕皮时,外表皮的大部分物质会被吸收。在蜕皮时,旧的表皮和上皮分离(此程序称为离解作用(apolysis)),催化蜕皮的液体酶被释放至旧表皮和上皮之间,同时透过消化内表皮(endocuticle)使外表皮(exocuticle)脱离,并将消化后的物质回收做为新表皮的原料。当新的表皮完全形成后,旧的上表皮和外表皮即会脱落[5]:16–20。
昆虫身体的四个主要部分是:背板(tergum)、胸板(Sternum),和两个侧板(pleura)。外骨骼上硬化扁平构造叫做骨片(sclerites),骨片可以再细分为背片(tergites)、腹片(sternites)和侧片(pleurites)[6]。
头节
[编辑]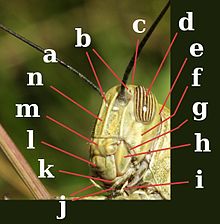

大部分昆虫的头皆包覆在坚硬且骨化的外骨骼头壳(head capsule)或上头壳(epicranium)之中,除了那些幼虫期没有完全骨化的物种,大部分是内生翅群;但就连无骨化或是稍微骨化的幼虫都倾向拥有完全骨化的上头壳,例如鞘翅目和膜翅目的幼虫,而环裂群(Cyclorrhapha)的幼虫则几乎没有头壳。
上头壳具有大部分的主要感觉器官,包括触角(antennae)、单眼(ocelli)和复眼(compound eyes)。口器也位于上头壳上。成虫的头壳不分节,但其实在胚胎期间头壳是由6个分节愈合而成,每节都拥有一对附肢,包括口器[7]。但现代的昆虫不是每节都有可见的附肢。
在昆虫众多的目之中,直翅目表现出最多的头部多样性,包括愈合处(sutures)和骨片(sclerites)。头顶(vertex)位于下口式(hypognathous)或是后口式(opisthognathous)之头型物种的复眼之间。在前口式(prognathous)的昆虫中,头顶不存在于复眼之间,而是常常在单眼附近。这是由于头部的主轴旋转了90°,才得以与身体的主轴平行。在某些物种中,这个部位有特殊的专有名词描述[8]:13。
The ecdysial suture is made of the coronal, frontal, and epicranial sutures plus the ecdysial and cleavage lines, which vary among different species of insects. The ecdysial suture is longitudinally placed on the vertex and separates the epicranial halves of the head to the left and right sides. Depending on the insect, the suture may come in different shapes: like either a Y, U, or V. Those diverging lines that make up the ecdysial suture are called the frontal or frontogenal sutures. Not all species of insects have frontal sutures, but in those that do, the sutures split open during ecdysis, which helps provide an opening for the new instar to emerge from the integument.
蜕皮缝(ecdysial suture)是由上面(coronal)缝、前面(frontal)缝、头上缝(epicranial suture)加上蜕皮线(ecdysial "line", 异于前述之ecdysial "suture")和裂线(cleavage line)组成,此缝在昆虫物种中呈现极大的相异性。蜕皮缝由顶部(vertex)往下纵向延伸并将上头壳(epicranial)分割成左右两半。不同的昆虫的蜕皮缝呈现不同形状,如Y形、U形或V形。这些组成蜕皮缝的切割线称为额缝(frontal suture)或是额颊缝(frontogenal suture)。并非所有的昆虫都拥有额缝,但是在那些具额缝的昆虫中,这些缝会在蜕皮时分裂剥离,提供一个开口帮助新一龄的昆虫从旧体壁中钻出。
The frons is that part of the head capsule that lies ventrad or anteriad of the vertex. The frons varies in size relative to the insect, and in many species the definition of its borders is arbitrary, even in some insect taxa that have well-defined head capsules. In most species, though, the frons is bordered at its anterior by the frontoclypeal or epistomal sulcus above the clypeus. Laterally it is limited by the fronto-genal sulcus, if present, and the boundary with the vertex, by the ecdysial cleavage line, if it is visible. If there is a median ocellus, it generally is on the frons, though in some insects such as many Hymenoptera, all three ocelli appear on the vertex. A more formal definition is that it is the sclerite from which the pharyngeal dilator muscles arise, but in many contexts that too, is not helpful.[7] In the anatomy of some taxa, such as many Cicadomorpha, the front of the head is fairly clearly distinguished and tends to be broad and sub-vertical; that median area commonly is taken to be the frons.[9]
额部(frons)是头壳的一部分,位于顶部的前面(anteriad)和腹面(ventrad)。额部的大小根据不同的昆虫物种而异,在许多物种中额部边界的定义并不明确,甚至是那些具有明确定义头壳的昆虫类群。在大部分的物种中,额部的边界在头楯(clypeus)上的额楯沟(frontoclypeal sulcus)或是口上沟(epistomal sulcus);而侧面的边界则是位于前额与颊之间的沟(fronto-genal sulcus);有些物种上部还有一条边界位于头顶(vertex)的蜕皮线(ecdysial cleavage line)。如果某物种具有中央单眼(median ocellus),通常位于额部上,然而,在某些物种上,如大多数的膜翅目,三颗单眼都位于头顶。比较正式的定义是 : 从咽扩张肌(pharyngeal dilator muscles)一路往上骨化,然而此定义在大部分情况没有太大的帮助[7]。在某些类群的解剖学中,如蝉下目(Cicadomorpha),头部的前面观构造可以清楚的分辨,而且倾向于近垂直的构造;于是中间的部分通常被视为额部[9]。

The clypeus is a sclerite between the face and labrum, which is dorsally separated from the frons by the frontoclypeal suture in primitive insects. The clypeogenal suture laterally demarcates the clypeus, with the clypeus ventrally separated from the labrum by the clypeolabral suture. The clypeus differs in shape and size, such as species of Lepidoptera with a large clypeus with elongated mouthparts. The cheek or gena forms the sclerotized area on each side of the head below the compound eyes extending to the gular suture. Like many of the other parts making up the insect's head, the gena varies among species, with its boundaries difficult to establish. For example, in dragonflies and damselflies, it is between the compound eyes, clypeus, and mouthparts. The postgena is the area immediately posteriad, or posterior or lower on the gena of pterygote insects, and forms the lateral and ventral parts of the occipital arch. The occipital arch is a narrow band forming the posterior edge of the head capsule arching dorsally over the foramen. The subgenal area is usually narrow, located above the mouthparts; this area also includes the hypostoma and pleurostoma.[8]:13–14 The vertex extends anteriorly above the bases of the antennae as a prominent, pointed, concave rostrum. The posterior wall of the head capsule is penetrated by a large aperture, the foramen. Through it pass the organ systems, such as nerve cord, esophagus, salivary ducts, and musculature, connecting the head with the thorax.[10]
头楯(clypeus)是脸部和上唇(labrum)之间的一个骨片(sclerite)。原始昆虫的头楯背面被额部上的额楯缝线(frontoclypeal suture)分割,而侧面的界线则是颊楯缝线(clypeogenal),腹面界线则是上唇的上唇楯缝线(clypeolabral suture)。不同的物种头楯大小形状相异,如鳞翅目具有较大的头楯和加长的口器。颊部(gena)在复眼下方头部两侧形成骨化的区块直至外咽片缝(gular suture)。如同其他组成昆虫头部的区块一般,颊部的外形因物种不同而异,而相异的情况族繁不及备载。
举例来说,蜻蛉目的颊部位于复眼(compound eyes)、头楯(clypeus)及口器(mouthparts)之间。在有翅亚纲的昆虫中,颊部后方或下方的区域称为后颊(postgena),且形成头弓(occipital arch)的侧边及腹边。头弓是一个狭带,形成头壳的后缘,且在背侧拱起盖过后头孔(foramen)。颊部之下的区域通常狭窄,位于口器之上。颊下区通常包含口下区(hypostoma)及口侧区(pleurostoma)[8]:13–14。头顶向前延伸至触角基部上方,形成一个突出的尖端,有凹面的突。头壳的后方穿过一个孔洞,也就是后头孔。从后头孔之间穿过的是器官系统,如神经节、食道(esophagus)、唾液管(salivary ducts)及连接头部与胸节的肌肉。[10]
On the posterior aspect of the head are the occiput, postgena, occipital foramen, posterior tentorial pit, gula, postgenal bridge, hypostomal suture and bridge, and the mandibles, labium, and maxilla. The occipital suture is well founded in species of Orthoptera, but not so much in other orders. Where found, the occipital suture is the arched, horseshoe-shaped groove on the back of the head, ending at the posterior of each mandible. The postoccipital suture is a landmark on the posterior surface of the head, and is typically near the occipital foremen. In pterygotes, the postocciput forms the extreme posterior, often U-shaped, which forms the rim of the head extending to the postoccipital suture. In pterygotes, such as those of Orthoptera, the occipital foramen and the mouth are not separated. The three types of occipital closures, or points under the occipital foramen that separate the two lower halves of the postgena, are: the hypostomal bridge, the postgenal bridge, and the gula. The hypostomal bridge is usually found in insects with hypognathous orientation. The postgenal bridge is found in the adults of species of higher Diptera and aculeate Hymenoptera, while the gula is found on some Coleoptera, Neuroptera, and Isoptera, which typically display prognathous-oriented mouthparts.[8]:15
头部的后方为后头区(occiput)、后颊、后头孔、后幕状骨孔(posterior tentorial pit)、口下缝(hypostomal suture)、口下接区(bridge)、大颚、下唇及小颚。直翅目的头缝线能被明显的观察到,其他目则非。头缝为头后方拱状、马蹄形的沟槽,收于大颚的后方。后头后缝(postoccipital suture)是区分头部后方的标线,通常位于后头孔附近。在有翅亚纲中,后头(postocciput)位于极后方,通常为U字形,通常形成头部延伸至后头后缝的边缘。在有翅亚纲中,例如直翅目,后头孔和口器相连。头部的闭合处有三种,也就是位于后头孔下方,将后颊区分为两个半区的点,分别为:口下带(hypostomal bridge)、后颊带(postgenal bridge)、外咽片(gula)。口下带通常可在下口式(hypognathous orientation)昆虫中发现,后颊带可以在大型双翅目成虫及膜翅目中的针尾下目(aculeate)成虫中发现,外咽片则可在鞘翅目、脉翅目、等翅下目(白蚁)等通常为前口式的昆虫中发现。[8]:15
复眼及单眼
[编辑]Most insects have one pair of large, prominent compound eyes composed of units called ommatidia (ommatidium, singular), possibly up to 30,000 in a single compound eye. This type of eye gives less resolution than eyes found in vertebrates, but it gives acute perception of movement. There can also be an additional two or three ocelli, which help detect low light or small changes in light intensity. The image perceived is a combination of inputs from the numerous ommatidia, located on a convex surface, thus pointing in slightly different directions. Compared with simple eyes, compound eyes possess very large view angles, and can detect fast movement and, in some cases, the polarization of light.[11]
大部分的昆虫都有一对主要的复眼(compound eye),复眼由小眼(ommatidia, 单数型:ommatidum)组成,一个复眼有可能由多达30000个小眼组成。这种形式的眼睛提供的分辨率相较于脊椎动物的眼睛来的低,但复眼能精准的感知移动的物种。同时,亦有可能生有2或3个单眼(ocelli),单眼能侦测低光源和微小的光密度变化。复眼感知到的影像是由无数个位于凸面上的小眼所输入的影像组成,因此昆虫能感知多个方向的影像。相较于单眼(simple eye),复眼的视线角度极大,且能感知快速的移动,甚至有些昆虫还能感知光的偏振(polarization)[11]。
Because the individual lenses are so small, the effects of diffraction impose a limit on the possible resolution that can be obtained (assuming they do not function as phased arrays). This can only be countered by increasing lens size and number. To see with a resolution comparable to our simple eyes, humans would require compound eyes that would each reach the size of their heads. Compound eyes fall into two groups: apposition eyes, which form multiple inverted images, and superposition eyes, which form a single erect image.[12][13] Compound eyes grow at their margins by the addition of new ommatidia.[14]
由于单一晶状体很小,衍射(diffraction)的作用限制了分辨率(假设它们作用方式与相位阵列(phased arrays)不同)。这个问题只能透过提升晶状体的大小和数量解决。如果要获得和人眼一样的分辨率,人类必须要生有和头颅同样大小的复眼。复眼能细分为两种,并置眼(apposition eyes)能输出复数个倒像,叠加眼(superposition eyes)能输出单个正像。复眼透过增加小眼数目来成长增大[14]。
触角
[编辑]
Antennae, sometimes called "feelers", are flexible appendages located on the insect's head which are used for sensing the environment. Insects are able to feel with their antennae because of the fine hairs (setae) that cover them.[15]:8–11 However, touch is not the only thing that antennae can detect; numerous tiny sensory structures on the antennae allow insects to sense smells, temperature, humidity, pressure, and even potentially sense themselves in space.[15]:8–11[16][17] Some insects, including bees and some groups of flies can also detect sound with their antennae.[18]
触角(antennae),有时也称为感觉器(feeler),为位于昆虫头部上具弹性的附肢,用以感知环境。昆虫之所以能利用触角感知环境是因为触角上面覆盖着细毛(刚毛, setae)[15]:8–11。然而,感知触觉不是触角的唯一功能;触角上所布满的无数受器使昆虫能够感知气味、温度、湿度、压力,甚至有可能感知他们本身的状态(本体感觉, 自我知觉)[15]:8–11[16][17]。有些昆虫,如蜜蜂和某些苍蝇,能够透过触角感知声音[18]。
The number of segments in an antenna varies considerably amongst insects, with higher flies having only 3-6 segments,[19] while adult cockroaches can have over 140.[20] The general shape of the antennae is also quite variable, but the first segment (the one attached to the head) is always called the scape, and the second segment is called the pedicel. The remaining antennal segments or flagellomeres are called the flagellum.[15]:8–11
触角部位的节数因虫而异,如Higher flies只有3-6节[19],蟑螂成虫却有超过140节[20]。触角的形状也非常歧异,但第一节(连结头部那节)永远都叫做柄节,第二节叫做梗节。剩下的部分或是鞭小节称为鞭节[15]:8–11。
General insect antenna types are shown below:
常见的昆虫触角样式如下:
![]() Aristate |
![]() Capitate |
![]() Clavate |
![]() Filiform |
![]() Flabellate |
![]() Geniculate |
![]() Setaceous |
![]() Lamellate |
![]() Moniliform |
![]() Pectinate |
![]() Plumose |
![]() Serrate |
![]() Stylate |
口器
[编辑]The insect mouthparts consist of the maxilla, labium, and in some species, the mandibles.[8]:16[21] The labrum is a simple, fused sclerite, often called the upper lip, and moves longitudinally, which is hinged to the clypeus. The mandibles (jaws) are a highly sclerotized pair of structures that move at right angles to the body, used for biting, chewing, and severing food. The maxillae are paired structures that can also move at right angles to the body and possess segmented palps. The labium (lower lip) is the fused structure that moves longitudinally and possesses a pair of segmented palps.[22]
昆虫的口器包括小颚(maxilla)、下唇(labium),在有些物种中也包含大颚(mandible)[8]:16[21]。上唇(labrum)是一片简单且愈合的骨片,可由头楯部 (clypeus)纵向拉动。大颚是一对高度骨化的结构,移动方向与身体垂直,用以啃咬、咀嚼和进食。小颚一对,移动方向也和身体垂直,并生有分节的附肢。下唇,是一个愈合的结构,纵向移动,并生有一对分节的附肢[22]。

Legend: a - antennae
c - compound eye
lb - labium
lr - labrum
md - mandibles
mx - maxillae
The mouthparts, along with the rest of the head, can be articulated in at least three different positions: prognathous, opisthognathous, and hypognathous. In species with prognathous articulation, the head is positioned vertically aligned with the body, such as species of Formicidae; while in a hypognathous type, the head is aligned horizontally adjacent to the body. An opisthognathous head is positioned diagonally, such as species of Blattodea and some Coleoptera.[23] The mouthparts vary greatly between insects of different orders, but the two main functional groups are mandibulate and haustellate. Haustellate mouthparts are used for sucking liquids and can be further classified by the presence of stylets, which include piercing-sucking, sponging, and siphoning. The stylets are needle-like projections used to penetrate plant and animal tissues. The stylets and the feeding tube form the modified mandibles, maxilla, and hypopharynx.[22]
口器和头部剩下的部位,能够被归类为至少三种型式:前口式(prognathous)、后口式(opisthognathous)与下口式(hypognathous)。下口式的物种头部和身体垂直,如蚁科(formicidae);前口式物种头部和身体平行排列;后口式物种头部和身体呈斜线,如蜚蠊目(Blattodea)和某些鞘翅目(Coleoptera)[23]。口器的种类因昆虫目的不同而异,但主要的两种为具颚式(mandibulate)和吸吮式(haustellate)。吸吮式口器用来吸取液体,并能以刺器(stylets)的有无进一步分类,分别为刺吸式(piercing-sucking)、汲取式(sponging)与虹吸式(siphoning)。刺器是一种针状的突起物,用以穿刺植物或是动物组织。刺器和取食管为特化的大颚、小颚与下咽(hypopharynx)[22]。
- Mandibulate mouthparts, among the most common in insects, are used for biting and grinding solid foods.
- Piercing-sucking mouthparts have stylets, and are used to penetrate solid tissue and then suck up liquid food.
- Sponging mouthparts are used to sponge and suck liquids, and lack stylets (e.g. most Diptera).
- Siphoning mouthparts lack stylets and are used to suck liquids, and are commonly found among species of Lepidoptera.
- 具颚式口器,用以啃咬与磨碎固体食物,大部分的昆虫皆为此类。 刺吸式口器,具刺器,以刺器刺穿固体组织后吸食液体食物。 汲取式口器,用以汲取与吸食液体,缺乏刺器,如大部分的双翅目(Diptera) 虹吸式口器,缺乏刺器,用以吸食液体,在大部分的鳞翅目(Lepidoptera)物种中被发现。
Mandibular mouthparts are found in species of Odonata, adult Neuroptera, Coleoptera, Hymenoptera, Blattodea, Orthoptera, and Lepidoptera. However, most adult Lepidoptera have siphoning mouthparts, while their larvae (commonly called caterpillars) have mandibles.
具颚式口器能在以下昆虫中被发现:蜻蛉目(Odonata)、脉翅目(Neuroptera)成虫、鞘翅目(Coleoptera)、膜翅目(Hymenoptera)、蜚蠊目(Blattodea)、直翅目(Orthoptera)、鳞翅目(Lepidoptera)。然而,鳞翅目成虫为虹吸式口器,它们的幼虫(通常被称为毛虫(caterpillar))为具颚式口器。
Mandibulate
[编辑]The labrum is a broad lobe forming the roof of the preoral cavity, suspended from the clypeus in front of the mouth and forming the upper lip.[1]:22–24 On its inner side, it is membranous and may be produced into a median lobe, the epipharynx, bearing some sensilla. The labrum is raised away from the mandibles by two muscles arising in the head and inserted medially into the anterior margin of the labrum. It is closed against the mandibles in part by two muscles arising in the head and inserted on the posterior lateral margins on two small sclerites, the tormae, and, at least in some insects, by a resilin spring in the cuticle at the junction of the labrum with the clypeus. [24] Until recently, the labrum generally was considered to be associated with first head segment. However, recent studies of the embryology, gene expression, and nerve supply to the labrum show it is innervated by the tritocerebrum of the brain, which is the fused ganglia of the third head segment. This is formed from fusion of parts of a pair of ancestral appendages found on the third head segment, showing their relationship.[1]:22–24 Its ventral, or inner, surface is usually membranous and forms the lobe-like epipharynx, which bears mechanosensilla and chemosensilla.[25][26]
具颚式
[编辑]上唇(labrum)是一覆盖于口前腔(preoral cavity)之上的宽叶,上接头楯(clypeus),并形成上唇(upper lip)[1]:22–24。上唇里边为膜质,且有可能形成一个中叶,也就是咽(epipharynx),生有一些感觉器。将上唇提起离开大颚的肌肉,为两条从头壳中生出,并插入上唇外缘中间的肌肉。而将上唇拉近大颚的肌肉,为两条从头壳生出,插入内缘侧边的两个小骨片上,也就是tormae,且,至少在某些昆虫中,被弹性蛋白(resilin)拉在上唇和头楯的关节处表皮上[24]。直到最近,上唇普遍被认为和第一头节有关。然而,根据最近的胚胎学、基因表现及上唇神经的研究,上唇由大脑的第三大脑(tritocerebrum)支配,第三大脑为第三头节的愈合神经结,且上唇为第三头节上古老附肢愈合的产物,此点也揭露了第三大脑和上唇的关联[1]:22–24。上唇的腹侧,也就是内侧,表面通常为膜质,且形成一个半叶状的咽(epipharynx),上头生有机械受器(mechanosensilla)及化学受器(chemosensilla)。
Chewing insects have two mandibles, one on each side of the head. The mandibles are positioned between the labrum and maxillae. The mandibles cut and crush food, and may be used for defense; generally, they have an apical cutting edge, and the more basal molar area grinds the food. They can be extremely hard (around 3 on Mohs, or an indentation hardness of about 30 kg/mm2); thus, many termites and beetles have no physical difficulty in boring through foils made from such common metals as copper, lead, tin, and zinc.[1]:22–24 The cutting edges are typically strengthened by the addition of zinc, manganese, or rarely, iron, in amounts up to about 4% of the dry weight.[25] They are typically the largest mouthparts of chewing insects, being used to masticate (cut, tear, crush, chew) food items. They open outwards (to the sides of the head) and come together medially. In carnivorous, chewing insects, the mandibles can be modified to be more knife-like, whereas in herbivorous chewing insects, they are more typically broad and flat on their opposing faces (e.g., caterpillars). In male stag beetles, the mandibles are modified to such an extent as to not serve any feeding function, but are instead are used to defend mating sites from other males. In ants, the mandibles also serve a defensive function (particularly in soldier castes). In bull ants, the mandibles are elongated and toothed, used as hunting (and defensive) appendages.
咀嚼式的昆虫拥有一对大颚,头部两侧各一。大颚位于上唇及小颚之间。大颚用以切割及磨碎食物,亦可用于防御;普遍来说,大颚有一个切面(cutting edge),与占大部分面积的磨面(molar area)用以磨碎食物。大颚的硬度可以极高(大约3摩氏硬度,或是30 kg/mm2的压痕硬度);因此,白蚁和甲虫在常见金属组成的土壤,如铜、铅、锡或锌[1]:22–24,挖掘不会遭受物理上的困难。切面通常被金属附加物强化,如锌与镁,较少见的还有铁,金属附加物占的比重大约是干重的4%[25]。大颚通常是咀嚼式昆虫口器中最大的部分,用以咀嚼(切、撕、粉碎、嚼)食物。大颚向外张开并向内靠拢。在肉食性的咀嚼式昆虫中,大颚可以被特化成刀状,然而在植食性昆虫中,大颚内侧通常宽扁(如毛虫)。在锹形虫雄虫中,大颚被特化且失去了取食功能,但能用来与其它雄虫争取配偶。在蚂蚁中,大颚也可用作防御用途(特别是兵蚁阶级)。在斗牛犬蚁中,大颚被延长且生有齿,用作猎食(和防御)。
Situated beneath the mandibles, paired maxillae manipulate food during mastication. Maxillae can have hairs and "teeth" along their inner margins. At the outer margin, the galea is a cupped or scoop-like structure, which sits over the outer edge of the labium. They also have palps, which are used to sense the characteristics of potential foods. The maxillae occupy a lateral position, one on each side of the head behind the mandibles. The proximal part of the maxilla consists of a basal cardo, which has a single articulation with the head, and a flat plate, the stipes, hinged to the cardo. Both cardo and stipes are loosely joined to the head by membrane so they are capable of movement. Distally on the stipes are two lobes, an inner lacinea and an outer galea, one or both of which may be absent. More laterally on the stipes is a jointed, leglike palp made up of a number of segments; in Orthoptera there are five. Anterior and posterior rotator muscles are inserted on the cardo, and ventral adductor muscles arising on the tentorium are inserted on both cardo and stipes. Arising in the stipes are flexor muscles of lacinea and galea and another lacineal flexor arises in the cranium, but neither the lacinea nor the galea has an extensor muscle. The palp has levator and depressor muscles arising in the stipes, and each segment of the palp has a single muscle causing flexion of the next segment.[24]
在大颚下方,成对的小颚在咀嚼时负责抓住食物。小颚内侧可能生有毛或是齿。在外侧,生有外叶(galea),外叶为杯状或汤匙状的结构,用以感受食物的存在。小颚也生有须,用来感受食物的存在。小颚位于侧面,大颚后方头部两侧各一。小颚靠近头部的一端基部生有轴节(cardo),轴节与头部以单关节相连,一个扁平板,也就是蝶绞节(stipes),生在轴节之上。轴节和蝶绞节都松弛的以膜质的结构连结头部,因此他们能够自由移动。在离头部的远端,蝶绞节之上生有两瓣,内侧为内叶(lacinea),外侧为外叶(galea),其中一者或是两者有可能消失。蝶绞节的外侧端生有具关节,足状的须,由数分节组成;直翅目为5节。前后侧的肌肉插入轴节中,而腹侧生于幕状骨(tentorium)上的收缩肌插入轴节和蝶绞节中。蝶绞节上生有外叶及内叶的屈肌,外叶的另一条屈肌着生于头壳,外叶及内叶皆不具有伸肌。小颚须有生于蝶绞节之上的提肌与升肌,且每一小颚须节皆具有一条肌肉,使下一节弯曲。[24]
In mandibulate mouthparts, the labium is a quadrupedal structure, although it is formed from two fused secondary maxillae. It can be described as the floor of the mouth. With the maxillae, it assists with manipulation of food during mastication or chewing or, in the unusual case of the dragonfly nymph, extends out to snatch prey back to the head, where the mandibles can eat it. The labium is similar in structure to the maxilla, but with the appendages of the two sides fused by the midline, so they come to form a median plate. The basal part of the labium, equivalent to the maxillary cardines and possibly including a part of the sternum of the labial segment, is called the postmentum. This may be subdivided into a proximal submentum and a distal mentum. Distal to the postmentum, and equivalent to the fused maxillary stipites, is the prementum. The prementum closes the preoral cavity from behind. Terminally, it bears four lobes, two inner glossae, and two outer paraglossae, which are collectively known as the ligula. One or both pairs of lobes may be absent or they may be fused to form a single median process. A palp arises from each side of the prementum, often being three-segmented.[24]
在具颚式口器中,下唇为一个四脚的结构,虽然他是从两个愈合的次要小颚(secondary maxillae)而来。可以用口器的地板来形容下唇,下唇用以在咀嚼的过程中把握住食物,但在一个不寻常的例子中,蜻蜓雉虫,能够向外延展以攫住猎物并拉向头部,再透过大颚进食。下唇的结构相似于小颚,但两边的须于中央愈合,因此形成一个中央平板。下唇的基部相当于小颚的轴节,且可能包含一部分的下唇节腹骨片,也就是下唇后基节(postmentum),有时可进一步细分为近端的下唇亚基节(submentum)及远端的下唇基节(mentum)。下唇亚基节的外端,是下唇前基节(prementum),相当于小颚的蝶绞节。下唇前基节盖住后方的口前腔(preoral cavity)。末端生有4个叶状体,一对内侧的中舌(glossa),及一对外侧的侧舌(paraglossa),合在一起称作唇舌(ligula)。一或二对叶状体可能消失或愈合形成一个中央突起。一个须着生于下唇前基节的两侧,通常为三节[24]。
The hypopharynx is a median lobe immediately behind the mouth, projecting forwards from the back of the preoral cavity; it is a lobe of uncertain origin, but perhaps associated with the mandibular segment;[24] in apterygotes, earwigs, and nymphal mayflies, the hypopharynx bears a pair of lateral lobes, the superlinguae (singular: superlingua). It divides the cavity into a dorsal food pouch, or cibarium, and a ventral salivarium into which the salivary duct opens.[1]:22–24 It is commonly found fused to the libium.[25] Most of the hypopharynx is membranous, but the adoral face is sclerotized distally, and proximally contains a pair of suspensory sclerites extending upwards to end in the lateral wall of the stomodeum. Muscles arising on the frons are inserted into these sclerites, which distally are hinged to a pair of lingual sclerites. These, in turn, have inserted into them antagonistic pairs of muscles arising on the tentorium and labium. The various muscles serve to swing the hypopharynx forwards and back, and in the cockroach, two more muscles run across the hypopharynx and dilate the salivary orifice and expand the salivarium.[24]
下咽(hypopharynx)为一个紧接在口后方的中央叶状体,从前口腔(preoral cavity)的后方往前突;此叶的起源目前未知,但推测与颚的小节有关[24];在无翅亚纲、蠼螋及蜉蝣雉虫中,下咽侧面生有一对叶状体,也就是舌上叶(superlinguae,单数形superlingua)。舌上叶将下咽划分为上下两腔,背侧的是食料腔(cibarium),腹侧则是连结到唾液管开口的唾液腔(salivarium)[1]。舌上叶经常被发现和下唇愈合[25]。下咽大部分为膜质,但接近口的那面末端骨化,且具有一对支撑用的骨片往上延伸连结至原口腔(stomodeum)侧壁的末端。从额部生出的肌肉也没入这些骨片中,同时末端连结一对舌骨片(lingual sclerites)。这些形成了多对在幕状骨和下唇拮抗的肌肉。这些多样的肌肉负责将下咽前后摆荡,且在蟑螂中,多了两条横跨下咽的肌肉,用以放大唾液口和扩大唾液腔[24]。
Piercing-sucking
[编辑]Mouthparts can have multiple functions. Some insects combine piercing parts along with sponging ones which are then used to pierce through tissues of plants and animals. Female mosquitoes feed on blood (hemophagous) making them disease vectors. The mosquito mouthparts consist of the proboscis, paired mandibles and maxillae. The maxillae form needle-like structures, called stylets, which are enclosed by the labium. When mosquito bites, maxillae penetrate the skin and anchor the mouthparts, thus allowing other parts to be inserted. The sheath-like labium slides back, and the remaining mouthparts pass through its tip and into the tissue. Then, through the hypopharynx, the mosquito injects saliva, which contains anticoagulants to stop the blood from clotting. And finally, the labrum (upper lip) is used to suck up the blood. Species of the genus Anopheles are characterized by their long palpi (two parts with widening end), almost reaching the end of labrum.[27]
刺吸式
[编辑]口器功能变化多端。有些昆虫结合了刺的部分和虹吸,用以穿刺植物或动物的组织。雌蚊的吸血性(hemophagous)使之成为病媒。蚊子的口器包含了食管(proboscis)、成对的大颚及小颚。小颚形成针状结构,称之为口针(stylets),收于下唇之内。当蚊子吸血时,小颚刺穿皮肤并固定口器,使其他部分得以植入。鞘状的下唇后缩,剩余的口器通过它的尖端并进入组织。接着,透过下咽,蚊子注入包含抗凝剂(anticoagulants)的唾液来阻止血液凝固,最后,透过上唇吸取血液。Anopheles 属的物种特化的小颚须(两末端宽大的部分)极长,几乎和上唇一样长[27]。
-
Horsefly (female)
Siphoning
[编辑]The proboscis is formed from maxillary galeae and is adaption found in some insects for sucking.[28] The muscles of the cibarium or pharynx are strongly developed and form the pump. In Hemiptera and many Diptera, which feed on fluids within plants or animals, some components of the mouthparts are modified for piercing, and the elongated structures are called stylets. The combined tubular structures are referred to as the proboscis, although specialized terminology is used in some groups.
食管由小颚外叶形成,且在某些昆虫中特化用以吸食用[28]。食料腔或咽的肌肉极度发达并形成泵。在取食植物或动物的液体的半翅目及许多双翅目中,口器的某些部分特化用以穿刺用,此延长的结构称为口针。此复合的管状结构称为食管,但在某些特定族群中有更特定的用词。
In species of Lepidoptera, it consists of two tubes held together by hooks and separable for cleaning. Each tube is inwardly concave, thus forming a central tube through which moisture is sucked. Suction is effected through the contraction and expansion of a sac in the head.[29] The proboscis is coiled under the head when the insect is at rest, and is extended only when feeding.[28] The maxillary palpi are reduced or even vestigial.[30] They are conspicuous and five-segmented in some of the more basal families, and are often folded.[8] The shape and dimensions of the proboscis have evolved to give different species wider and therefore more advantageous diets.[28] There is an allometric scaling relationship between body mass of Lepidoptera and length of proboscis[31] from which an interesting adaptive departure is the unusually long-tongued hawk moth Xanthopan morganii praedicta. Charles Darwin predicted the existence and proboscis length of this moth before its discovery based on his knowledge of the long-spurred Madagascan star orchid Angraecum sesquipedale.[32]
在鳞翅目中,食管由两个由钩连接的管组成,且可分离彼此以便清洁。管巢内的部分生有沟渠,因此形成一个中央管道,液体从之经过。吸取的过程与液体浓度及头部中一个囊的舒张有关[29]。在休息时,食管卷起收于头部下方,并只在取食时张开[28]。小颚须退化甚至只剩下痕迹[30]。在某些基群中,小颚须明显且分为五节,且通常卷起[8]。食管的形状及尺寸因不同的物种而异,因此对各自的食性有益[28]。鳞翅目身体重量和食管长度为具缩放关系的异速成长[31],特别是一个适应性偏差的案例:Xanthopan morganii praedicta,长喙天蛾。查尔斯‧达尔文(Charles Darwin)根据他对长花萼的马达加斯加树兰 Angraecum sesquipedale的研究,预测了此种天蛾的存在与其食管的长度。
Sponging
[编辑]The mouthparts of insects that feed on fluids are modified in various ways to form a tube through which liquid can be drawn into the mouth and usually another through which saliva passes. The muscles of the cibarium or pharynx are strongly developed to form a pump.[24] In nonbiting flies, the mandibles are absent and other structures are reduced; the labial palps have become modified to form the labellum, and the maxillary palps are present, although sometimes short. In Brachycera, the labellum is especially prominent and used for sponging liquid or semiliquid food.[33] The labella are a complex structure consisting of many grooves, called pseudotrachea, which sops up liquids. Salivary secretions from the labella assist in dissolving and collecting food particles so they can be more easily taken up by the pseudotracheae; this is thought to occur by capillary action. The liquid food is then drawn up from the pseudotracheae through the food channel into the esophagus.[34]
汲取式
[编辑]取食液体的昆虫口器变化多端,形成一个管道让流质食物通过,且通常有另一个管道让唾液通过。食料腔或咽的肌肉极度发达形成一个泵[24]。在不咬人的苍蝇中,大颚消失且其他结构退化;下唇须特化形成唇瓣(labellum),且小颚须存在,虽然有时候可能很短。在短角亚目(Brachycera)中,唇特别重要,用以汲取液体或半液体食物[33]。唇瓣(labella)为一包含数个沟渠的复杂结构,此沟渠称为假器管(pseudotrachea),也就是吸取液体的部位。从唇瓣分泌的唾液可以帮助溶解并收集食物颗粒,使得他们能更容易被假器管吸取;此行为被认为由毛细作用所驱动。液体食物被假器管吸入后通过食料腔进入食道(esophagus)[34]。
The mouthparts of bees are of a chewing and lapping-sucking type. Lapping is a mode of feeding in which liquid or semiliquid food adhering to a protrusible organ, or "tongue", is transferred from substrate to mouth. In the honey bee (Hymenoptera: Apidae: Apis mellifera), the elongated and fused labial glossae form a hairy tongue, which is surrounded by the maxillary galeae and the labial palps to form a tubular proboscis containing a food canal. In feeding, the tongue is dipped into the nectar or honey, which adheres to the hairs, and then is retracted so the adhering liquid is carried into the space between the galeae and labial palps. This back-and-forth glossal movement occurs repeatedly. Movement of liquid to the mouth apparently results from the action of the cibarial pump, facilitated by each retraction of the tongue pushing liquid up the food canal.[1]:22–24
蜜蜂的口器为咀吸式。吸为一种取食液体或半液体食物的方式,将食物吸附在一个可伸缩的器官上,也就是舌(tongue),最后送入口中。在西方蜂(Hymenoptera: Apidae: Apis mellifera)中,延长且愈合的中舌(glossae )形成一根毛茸茸的舌,外层被小颚外叶及小颚须包覆,形成一个管状的食管,内含一个食物通道。取食时,舌浸入花粉或蜂蜜中,以毛吸附之,然后缩回舌让吸附的液体进入到外叶与小颚须之间的空间中。中舌会持续前后来回,形成一个抽取食物的泵,每次舌的收回都可以帮助将食物推入食料腔中[1]:22–24。
Thorax
[编辑]The insect thorax has three segments: the prothorax, mesothorax, and metathorax. The anterior segment, closest to the head, is the prothorax; its major features are the first pair of legs and the pronotum. The middle segment is the mesothorax; its major features are the second pair of legs and the anterior wings, if any. The third, the posterior, thoracic segment, abutting the abdomen, is the metathorax, which bears the third pair of legs and the posterior wings. Each segment is dilineated by an intersegmental suture. Each segment has four basic regions. The dorsal surface is called the tergum (or notum) to distinguish it from the abdominal terga.[1]:22–24 The two lateral regions are called the pleura (singular: pleuron), and the ventral aspect is called the sternum. In turn, the notum of the prothorax is called the pronotum, the notum for the mesothorax is called the mesonotum and the notum for the metathorax is called the metanotum. Continuing with this logic, there is also the mesopleura and metapleura, as well as the mesosternum and metasternum.[8]
胸节
[编辑]昆虫的胸节分三节:前胸(prothorax)、中胸(mesothorax)及后胸(metathorax),前侧最靠近头部的分节为前胸;前胸的特征为第一对足及前胸背板(pronotum)。中央的分节为中胸;特征为第二对足及前翅(如果具翅)。位于后侧的第三节为后胸,生有第三对足及后翅。各节之间可被缝线区隔。每节又可分为四个基本区域,背侧称作背板(tergum 或notum),腹节的背板则称作腹背板(terga)[1]:22–24。两侧边区域则称作侧板(pleura,单数形为pleuron ),腹侧区域则称作腹板(sternum)。依此类推,前胸的背板称作前胸背板,中胸,中胸背板,后胸则是后胸背板。照此规则,中胸侧板、后胸侧板、中胸腹板、后胸腹板[8]。
The tergal plates of the thorax are simple structures in apterygotes and in many immature insects, but are variously modified in winged adults. The pterothoracic nota each have two main divisions: the anterior, wing-bearing alinotum and the posterior, phragma-bearing postnotum. Phragmata (singular: phragma) are plate-like apodemes that extend inwards below the antecostal sutures, marking the primary intersegmental folds between segments; phragmata provide attachment for the longitudinal flight muscles. Each alinotum (sometimes confusingly referred to as a "notum") may be traversed by sutures that mark the position of internal strengthening ridges, and commonly divides the plate into three areas: the anterior prescutum, the scutum, and the smaller posterior scutellum. The lateral pleural sclerites are believed to be derived from the subcoxal segment of the ancestral insect leg. These sclerites may be separate, as in silverfish, or fused into an almost continuous sclerotic area, as in most winged insects.[1]:22–24
胸背板在无翅亚纲和许多非成虫中结构简易,但在有翅成虫中则多样的特化。生翅胸(pterothoracic )的背板则区分为两区,前方为生有翅的翅背板(alinotum ),后方则为生有悬骨(phragma)的后背板(postnotum)。悬骨(Phragmata ,单数形为phragma)为脊前缝线(antecostal suture)下内伸的板状游离骨(apodemes),悬骨区分节与节;悬骨提供纵走飞翔肌(longitudinal flight muscle)附着的部位。翅背板(alinotum 有时会与背板,notum,混淆),有几条横走缝线,这些缝线代表内部的强化棱脊所在的位置,且常将翅背板分为三区,前侧为前楯片(prescutum),中间为楯片(scutum),最小位于后侧的为小楯片(scutellum)。侧面的侧骨片被认为是从古老昆虫足的亚基节衍生而来。三骨片可能分离,如衣鱼;亦可能愈合为一连续的骨片区,如大部分的有翅昆虫。[1]:22–24
Prothorax
[编辑]The prothorax on this conehead is shielded by a large pronotum that extends from over the cervix (neck) on the anterior side to cover the mesothorax and most of the metathorax on the posterior side, as well as covering the pleura on the prothorax.[8] The pronotum of the prothorax may be simple in structure and small in comparison with the other nota, but in beetles, mantids, many bugs, and some Orthoptera, the pronotum is expanded, and in cockroaches, it forms a shield that covers part of the head and mesothorax.[1]:22–24
前胸
[编辑]前胸被大面积的前胸背板盖住,由颈部(cervix )延伸盖住中胸及大部分的后胸,也盖住了前胸的侧板[8]。前胸背板相较于其他的胸节背板可能小且结构简单,但在甲虫、螳螂、许多臭虫及某些直翅目中,前胸背板延展,且在蟑螂中,前胸背板形成一个盖住部分头部和中胸的构造[1]:22–24。
Pterothorax
[编辑]Because the mesothorax and metathorax hold the wings, they have a combined name called the pterothorax (pteron = wing). The forewing, which goes by different names in different orders (e.g., the tegmina in Orthoptera and elytra in Coleoptera), arises between the mesonotum and the mesopleura, and the hindwing articulates between the metanotum and metapleura. The legs arise from the mesopleura and metapleura. The mesothorax and metathorax each have a pleural suture (mesopleural and metapleural sutures) that runs from the wing base to the coxa of the leg. The sclerite anterior to the pleural suture is called the episternum (serially, the mesepisternum and metepisternum). The sclerite posterior to the suture is called the epimiron (serially, the mesepimiron and metepimiron). Spiracles, the external organs of the respiratory system, are found on the pterothorax, usually one between the pro- and mesopleoron, as well as one between the meso- and metapleuron.[8]
生翅胸
[编辑]由于翅膀生于中胸及后胸上,因此也可称作为生翅胸(pterothorax,pteron=wing)。前翅(forewing)在不同的目中有不同的名字(直翅目:翅覆,鞘翅目:翅鞘),着生于中胸背板及中胸侧板之间,而后翅的关节则着生于后胸背板及后胸侧板之间。足着生于中胸侧板及后胸背板之间。中胸和后胸各有一从翅膀基部延伸至足基节的侧板缝线(pleural suture,中胸侧板缝线及后胸侧板缝线)。位于侧板缝线前侧的骨片称作前侧片(episternum )(中胸前侧片mesepisternum 、后胸前侧片metepisternum),位于侧板缝线后方的骨片称作后侧片(epimiron )(中胸后侧片mesepimiron 、后胸后侧片metepimiron)。气孔,呼吸系统的外部器官,能在生翅胸上发现,通常在前-中侧板及中-后侧板之间各一[8]。
The ventral view or sternum follows the same convention, with the prosternum under the prothorax, the mesosternum under the mesothorax and the metasternum under the metathorax. The notum, pleura, and sternum of each segment have a variety of different sclerites and sutures, varying greatly from order to order, and they will not be discussed in detail in this section.[8]
腹侧,也就是腹板也遵循同样规律,前胸腹板、中胸腹板及后胸腹板。每个体节的背板、侧板及腹板各有多样的骨片及缝线,依目的不同而变化多端,在本节中将不详细讨论[8]。
Wings
[编辑]Most phylogenetically advanced insects have two pairs of wings located on the second and third thoracic segments.[1]:22–24 Insects are the only invertebrates to have developed flight capability, and this has played an important part in their success. Insect flight is not very well understood, relying heavily on turbulent aerodynamic effects. The primitive insect groups use muscles that act directly on the wing structure. The more advanced groups making up the Neoptera have foldable wings, and their muscles act on the thorax wall and power the wings indirectly.[1]:22–24 These muscles are able to contract multiple times for each single nerve impulse, allowing the wings to beat faster than would ordinarily be possible.
Insect flight can be extremely fast, maneuverable, and versatile, possibly due to the changing shape, extraordinary control, and variable motion of the insect wing. Insect orders use different flight mechanisms; for example, the flight of a butterfly can be explained using steady-state, nontransitory aerodynamics, and thin airfoil theory.
翅膀
[编辑]大多数在系统发生学上先进的昆虫都具有两对翅膀,方别位于中胸及后胸。昆虫是唯一发展出飞行能力的无脊椎动物,这点为昆虫的成功贡献了极大的部分。关于昆虫的飞行尚未完全了解,只知道其极度依赖紊流空气效应( turbulent aerodynamic effects)。原始的昆虫类群用肌肉直接驱动翅膀。较为后期的类群,新翅类群(Neoptera)具有可折叠的翅膀,且他们的肌肉施力于胸壁上,间接驱动翅膀[1]:22–24。这些肌肉能够在一次的神经冲动内收缩好几次,使翅膀能够挥动的比正常预期得更快。昆虫飞行可以极为快速、机动及多种功能,可能是因为持续变化的形状、超常的控制及多样的动作方式。不同的目拥有不同的飞行机制;例如,蝴蝶的飞行可以借由稳定态、非暂态性的空气动力学及薄翼理论来解释。

Internal
[编辑]Each of the wings consists of a thin membrane supported by a system of veins. The membrane is formed by two layers of integument closely apposed, while the veins are formed where the two layers remain separate and the cuticle may be thicker and more heavily sclerotized. Within each of the major veins is a nerve and a trachea, and, since the cavities of the veins are connected with the hemocoel, hemolymph can flow into the wings.[24] Also, the wing lumen, being an extension of the hemocoel, contains the tracheae, nerves, and hemolymph. As the wing develops, the dorsal and ventral integumental layers become closely apposed over most of their area, forming the wing membrane. The remaining areas form channels, the future veins, in which the nerves and tracheae may occur. The cuticle surrounding the veins becomes thickened and more heavily sclerotized to provide strength and rigidity to the wing. Hairs of two types may occur on the wings: microtrichia, which are small and irregularly scattered, and macrotrichia, which are larger, socketed, and may be restricted to veins. The scales of Lepidoptera and Trichoptera are highly modified macrotrichia.[25]
内部
[编辑]翅膀由以翅脉作骨架的薄膜构成。薄膜由两层极接近的外皮堆叠而成,而翅脉存在于两外皮之间的空间,翅脉位置的表皮可能较厚且高度骨化。主要的脉之中具有神经及气管(trachea),且由于翅脉中的空间与循环系统相连,血淋巴能够流入翅膀中[24]。翅腔(wing lumen)为循环系统的一部分,内含气管、神经及血淋巴。随着翅膀的发育,腹侧及背侧的外皮大部分的面积会慢慢靠近彼此,形成翅膜。其他的区域则形成管道,也就是未来的翅脉,同时也是气管及神经生长之处。翅脉周遭的表皮加厚且高度骨化,提供翅膀力量及刚度。翅膀上可能生有两种毛发:微毛(microtrichia),小且不规则分散,大毛(macrotrichia),大且着生于槽中,可能只生长于脉上。鳞翅目及毛翅目的鳞片为高度特化的大毛[25]。
Veins
[编辑]
In some very small insects, the venation may be greatly reduced. In chalcid wasps, for instance, only the subcosta and part of the radius are present. Conversely, an increase in venation may occur by the branching of existing veins to produce accessory veins or by the development of additional, intercalary veins between the original ones, as in the wings of Orthoptera (grasshoppers and crickets). Large numbers of cross-veins are present in some insects, and they may form a reticulum as in the wings of Odonata (dragonflies and damselflies) and at the base of the forewings of Tettigonioidea and Acridoidea (katydids and grasshoppers, respectively).[24]
翅脉
[编辑]翅脉是根据Comstock-Needham系统来命名。在一些极小的昆虫中,翅脉有可能极度缩减,例如,在小蜂总科中(chalcid wasps),只有亚前缘脉(subcosta ,Sc)及部分的径脉(raidus,R)存在。相反地,翅脉有可能增加,可能透过原有翅脉的分支以形成副脉(accessory veins),或是透过新翅脉的增生,形成介于原有翅脉之间的插脉(intercalary veins),例如直翅目的翅膀。大量的横脉存在于一些昆虫中,并分别于蜻蛉目的翅及螽斯科(Tettigonioidea)与蝗虫总科(Acridoidea)的前翅基部形成网状结构(reticulum)[24]。
The archedictyon is the name given to a hypothetical scheme of wing venation proposed for the very first winged insect. It is based on a combination of speculation and fossil data. Since all winged insects are believed to have evolved from a common ancestor, the archediction represents the "template" that has been modified (and streamlined) by natural selection for 200 million years. According to current dogma, the archedictyon contained six to eight longitudinal veins. These veins (and their branches) are named according to a system devised by John Comstock and George Needham—the Comstock-Needham system:[35]
理想翅脉(archedictyon )系指假想的,第一只有翅昆虫的翅脉。基于推测及化石资料而成。由于普遍相信所有的有翅昆虫都是由一共同祖先演化而来,因此理想翅脉扮演的是一个模板,代表着经过2亿年天择后特化(和优化)的翅的雏形。根据现时公认的理想翅脉,共包含6至8条纵走脉。这些脉(及他们的分支)以John Comstock及George Needham所设立的系统所命名,Comstock-Needham system系统[35]:
- Costa (C) - the leading edge of the wing
- Subcosta (Sc) - second longitudinal vein (behind the costa), typically unbranched
- Radius (R) - third longitudinal vein, one to five branches reach the wing margin
- Media (M) - fourth longitudinal vein, one to four branches reach the wing margin
- Cubitus (Cu) - fifth longitudinal vein, one to three branches reach the wing margin
- Anal veins (A1, A2, A3) - unbranched veins behind the cubitus
- 前缘脉(Costa (C)) - 翅的上缘 亚前缘脉(Subcosta (Sc)) - 第二条纵走脉(前缘脉之后),通常不分支 径脉(Radius (R)) - 第三条纵走脉,1到5条延伸至翅末端的分支 中脉(Media (M)) - 第四条纵走脉,1到4条延伸至翅末端的分支 肘脉(Cubitus (Cu)) - 第五条纵走脉,1到3条延伸至翅末端的分支 臀脉(Anal veins (A1, A2, A3)) - 肘脉后一条不分支的脉
The costa (C) is the leading marginal vein on most insects, although a small vein, the precosta, is sometimes found above the costa. In almost all extant insects,[1]:41–42 the precosta is fused with the costa; the costa rarely ever branches because it is at the leading edge, which is associated at its base with the humeral plate. The trachea of the costal vein is perhaps a branch of the subcostal trachea. Located after the costa is the third vein, the subcosta, which branches into two separate veins: the anterior and posterior. The base of the subcosta is associated with the distal end of the neck of the first axillary. The fourth vein is the radius, which is branched into five separate veins. The radius is generally the strongest vein of the wing. Toward the middle of the wing, it forks into a first undivided branch (R1) and a second branch, called the radial sector (Ra), which subdivides dichotomously into four distal branches (R2, R3, R4, R5). Basally, the radius is flexibly united with the anterior end of the second axillary (2Ax).[36]
前缘脉为大多数昆虫的上缘,虽然有一条小脉,先前缘脉(precosta),有时候位置高于前缘脉。在大多数现生的昆虫中[1]:41–42,先前缘脉是和前缘脉愈合的;由于基部在肩板(humeral plate)的位置,前缘脉位于上缘,因此通常不分支。前缘脉的气管可能是亚前缘脉气管的分支。位于前缘脉之后的是第三条脉,也就是亚前缘脉,又分支成2条独立的脉:前亚前缘脉(anterior)及后亚前缘脉(posterior)。亚前缘脉的基部与第一翅腱骨的远端颈部有关。第四条脉是径脉,又分支成5条独立的脉。径脉通常是最强壮的翅脉。往翅的中央延伸,分成一条不可细分的分支(R1)及一条径区脉(radial sector,Ra),又可两两细分成4条位于远端的脉(R2, R3, R4, R5)。径脉的基部弹性的生于第二翅腱骨(2Ax)前侧的末端[36]。
The fifth vein of the wing is the media. In the archetype pattern (A), the media forks into two main branches, a media anterior (MA), which divides into two distal branches (MA1, MA2), and a median sector, or media posterior (MP), which has four terminal branches (M1, M2, M3, M4). In most modern insects, the media anterior has been lost, and the usual "media" is the four-branched media posterior with the common basal stem. In the Ephemerida, according to present interpretations of the wing venation, both branches of the media are retained, while in Odonata, the persisting media is the primitive anterior branch. The stem of the media is often united with the radius, but when it occurs as a distinct vein, its base is associated with the distal median plate (m') or is continuously sclerotized with the latter. The cubitus, the sixth vein of the wing, is primarily two-branched. The primary forking takes place near the base of the wing, forming the two principal branches (Cu1, Cu2). The anterior branch may break up into a number of secondary branches, but commonly it forks into two distal branches. The second branch of the cubitus (Cu2) in Hymenoptera, Trichoptera, and Lepidoptera, was mistaken by Comstock and Needham for the first anal. Proximally, the main stem of the cubitus is associated with the distal median plate (m') of the wing base.[36]
第五条脉是中脉。在理想翅脉图中,中脉分支成二个主要的分支,前中脉(MA),又可细分成二末端的脉(MA1, MA2),另一主分支为中区脉,也就是后中脉(MP),又可细分成4个末端的脉(M1, M2, M3, M4)。在大多数现代昆虫中,前中脉都已经丢失,而通常所谓"中脉"是基于一共同基脉且分四叉的后中脉。在蜉蝣目中,根据现生翅脉的分析,中脉的两条分支都还存在,然而在蜻蛉目中,还存在的中脉是原始前中脉的分支。中脉的干常常和径脉愈合,但当它是一条独立的脉时,中脉的基部位于中板(median plate)的远端,且两者以骨化连接[36]。
The postcubitus (Pcu) is the first anal of the Comstock and Needham system. The postcubitus, however, has the status of an independent wing vein and should be recognized as such. In nymphal wings, its trachea arises between the cubital trachea and the group of vannal tracheae. In the mature wings of more generalized insects, the postcubitus is always associated proximally with the cubitus, and is never intimately connected with the flexor sclerite (3Ax) of the wing base. In Neuroptera, Mecoptera, and Trichoptera, the postcubitus may be more closely associated with the vannal veins, but its base is always free from the latter. The postcubitus is usually unbranched; primitively, it is two-branched. The vannal veins (lV to nV) are the anal veins immediately associated with the third axillary, and which are directly affected by the movement of this sclerite that brings about the flexion of the wings. In number, the vannal veins vary from one to 12, according to the expansion of the vannal area of the wing. The vannal tracheae usually arise from a common tracheal stem in nymphal insects, and the veins are regarded as branches of a single anal vein. Distally, the vannal veins are either simple or branched. The jugal vein (J) of the jugal lobe of the wing is often occupied by a network of irregular veins, or it may be entirely membranous; sometimes it contains one or two distinct, small veins, the first jugal vein, or vena arcuata, and the second jugal vein, or vena cardinalis (2J).[36]
第六条脉,肘脉,在原始的情况分两支。原始的分支位于翅膀基部附近,形成2条主要的分支(Cu1, Cu2)。前分支可能进一步分成大量的次级分支,但通常只分支成二远端的分支。第二条主要的分支(Cu2)在膜翅目、毛翅目及鳞翅目中,被Comstock和Needham错当成第一臀脉。肘脉的主干大约连结于中板的远端。后肘脉在Comstock - Needham系统中是第一臀脉。然而,后肘脉具有独立一条脉的地位,也应被当作独立的一条脉。在若虫的翅膀中,气管生于肘脉气管与扇气管群之间。在比较普遍的昆虫的成熟翅膀中,后肘脉永远着生于肘脉之上,不和第三翅腱骨连接。在脉翅目、广翅目、毛翅目中,后肘脉可能更靠近扇脉,但基部永远不与扇脉相连。后肘脉通常不分支;但在原始的情况中,后肘脉分二支。扇脉(lV to nV),为最后的脉,着生于第三翅腱骨上,第三翅腱骨的移动直接影响扇脉,并造成翅膀的弯曲。扇脉的数量分布由1至12,依扇区的面积而异。扇气管通常由若虫的气管主干衍生,且扇脉群被认为是单一臀脉的分支。扇脉群的远端,可能简单或复杂分支。垂脉(J)可能被不规则脉的网络覆盖,亦可能完全膜质;有时候包含一或二条明显的小脉,也就是第一垂脉,或称为弓状脉(vena arcuata),第二垂脉,也称为韧脉(vena cardinalis)[36]。
- C-Sc cross-veins - run between the costa and subcosta
- R cross-veins - run between adjacent branches of the radius
- R-M cross-veins - run between the radius and media
- M-Cu cross-veins - run between the media and cubitus
- C-Sc横脉 - 跨过前缘脉及亚前缘脉 R横脉 - 跨过径脉分支群 R-M横脉 - 跨过径脉及中脉 M-Cu横脉 - 跨过中脉及肘脉
All the veins of the wing are subject to secondary forking and to union by cross-veins. In some orders of insects, the cross-veins are so numerous, the whole venational pattern becomes a close network of branching veins and cross-veins. Ordinarily, however, a definite number of cross-veins having specific locations occurs. The more constant cross-veins are the humeral cross-vein (h) between the costa and subcosta, the radial cross-vein (r) between R and the first fork of Rs, the sectorial cross-vein (s) between the two forks of R8, the median cross-vein (m-m) between M2 and M3, and the mediocubital cross-vein (m-cu) between the media and the cubitus.[36]
所有的翅脉皆为次级分叉且被横脉串联。在某些目的昆虫中,横脉非常大量,整个脉相变成一个由分脉及横脉交织成的网络。然而通常而言,横脉发生的数量和位置有关。较密集的横脉是位于前缘脉及亚前缘脉之间的肩横脉(h),径横脉(r)位于R脉及Rs脉的第一条分支之间,有关该区域的横脉位于R8脉的二分支之间,中横脉(m-m)位于M2及M3之间,中肘横脉(m-cu)位于中脉及肘脉之间[36]。
The veins of insect wings are characterized by a convex-concave placement, such as those seen in mayflies (i.e., concave is "down" and convex is "up"), which alternate regularly and by their branching; whenever a vein forks there is always an interpolated vein of the opposite position between the two branches. The concave vein will fork into two concave veins (with the interpolated vein being convex) and the regular alteration of the veins is preserved.[37] The veins of the wing appear to fall into an undulating pattern according to whether they have a tendency to fold up or down when the wing is relaxed. The basal shafts of the veins are convex, but each vein forks distally into an anterior convex branch and a posterior concave branch. Thus, the costa and subcosta are regarded as convex and concave branches of a primary first vein, Rs is the concave branch of the radius, posterior media the concave branch of the media, Cu1 and Cu2 are respectively convex and concave, while the primitive postcubitus and the first vannal have each an anterior convex branch and a posterior concave branch. The convex or concave nature of the veins has been used as evidence in determining the identities of the persisting distal branches of the veins of modern insects, but it has not been demonstrated to be consistent for all wings.[24][36]
昆虫翅脉成凹凸状,如同在蜉蝣目中所见一般,规律的连同分支一起凹凸分布;每当一个脉分叉成二时永远会有一个逆位的脉插入两分支间。一下凹的脉会分叉成二下凹的脉(之间插入一上凸的脉),规律的凹凸交替也存在[37]。无论休息时翅膀是立起或平放的,翅脉都成波浪状的样式。翅脉的基轴是上凸的,但每条脉往远端分叉,形成一上凸的前分支及一下凹的后分支。因此,前缘脉及亚前缘脉被认为是原始第一翅脉的上凸及下凹分支,Rs是径脉的下凹分支,后中脉是中脉的下凹分支,Cu1及Cu2分别为上凸及下凹,然而原始的后肘脉及第一扇脉各拥有一前上凸分支及一后下凹分支。上凸及下凹的性质被用作证据,以辨别现生昆虫翅脉远端的分支,但并不是所有的翅膀都遵循本规律[24][36]。
Fields
[编辑]
Wing areas are delimited and subdivided by fold lines, along which the wings can fold, and flexion lines, which flex during flight. Between the flexion and the fold lines, the fundamental distinction is often blurred, as fold lines may permit some flexibility or vice versa. Two constants, found in nearly all insect wings, are the claval (a flexion line) and jugal folds (or fold line), forming variable and unsatisfactory boundaries. Wing foldings can very complicated, with transverse folding occurring in the hindwings of Dermaptera and Coleoptera, and in some insects, the anal area can be folded like a fan.[1]:41–42 The four different fields found on insect wings are:
- Remigium
- Anal area (vannus)
- Jugal area
- Axillary area
- Alula
Most veins and cross-veins occur in the anterior area of the remigium, which is responsible for most of the flight, powered by the thoracic muscles. The posterior portion of the remigium is sometimes called the clavus; the two other posterior fields are the anal and jugal areas.[1]:41–42 When the vannal fold has the usual position anterior to the group of anal veins, the remigium contains the costal, subcostal, radial, medial, cubital, and postcubital veins. In the flexed wing, the remigium turns posteriorly on the flexible basal connection of the radius with the second axillary, and the base of the mediocubital field is folded medially on the axillary region along the plica basalis (bf) between the median plates (m, m') of the wing base.[36]
The vannus is bordered by the vannal fold, which typically occurs between the postcubitus and the first vannal vein. In Orthoptera, it usually has this position. In the forewing of Blattidae, however, the only fold in this part of the wing lies immediately before the postcubitus. In Plecoptera, the vannal fold is posterior to the postcubitus, but proximally it crosses the base of the first vannal vein. In the cicada, the vannal fold lies immediately behind the first vannal vein (lV). These small variations in the actual position of the vannal fold, however, do not affect the unity of action of the vannal veins, controlled by the flexor sclerite (3Ax), in the flexion of the wing. In the hindwings of most Orthoptera, a secondary vena dividens forms a rib in the vannal fold. The vannus is usually triangular in shape, and its veins typically spread out from the third axillary like the ribs of a fan. Some of the vannal veins may be branched, and secondary veins may alternate with the primary veins. The vannal region is usually best developed in the hindwing, in which it may be enlarged to form a sustaining surface, as in Plecoptera and Orthoptera. The great fan-like expansions of the hindwings of Acrididae are clearly the vannal regions, since their veins are all supported on the third axillary sclerites on the wing bases, though Martynov (1925) ascribes most of the fan areas in Acrididae to the jugal regions of the wings. The true jugum of the acridid wing is represented only by the small membrane (Ju) mesad of the last vannal vein. The jugum is more highly developed in some other Orthoptera, as in the Mantidae. In most of the higher insects with narrow wings, the vannus becomes reduced, and the vannal fold is lost, but even in such cases, the flexed wing may bend along a line between the postcubitus and the first vannal vein.[36]
The jugal region, or neala, is a region of the wing that is usually a small membranous area proximal to the base of the vannus strengthened by a few small, irregular vein-like thickenings; but when well developed, it is a distinct section of the wing and may contain one or two jugal veins. When the jugal area of the forewing is developed as a free lobe, it projects beneath the humeral angle of the hindwing and thus serves to yoke the two wings together. In the Jugatae group of Lepidoptera, it bears a long finger-like lobe. The jugal region was termed the neala ("new wing") because it is evidently a secondary and recently developed part of the wing.[36]
The auxiliary region containing the axillary sclerites has, in general, the form of a scalene triangle. The base of the triangle (a-b) is the hinge of the wing with the body; the apex (c) is the distal end of the third axillary sclerite; the longer side is anterior to the apex. The point d on the anterior side of the triangle marks the articulation of the radial vein with the second axillary sclerite. The line between d and c is the plica basalis (bf), or fold of the wing at the base of the mediocubital field.[36]
At the posterior angle of the wing base in some Diptera there is a pair of membranous lobes (squamae, or calypteres) known as the alula. The alula is well developed in the house fly. The outer squama (c) arises from the wing base behind the third axillary sclerite (3Ax) and evidently represents the jugal lobe of other insects (A, D); the larger inner squama (d) arises from the posterior scutellar margin of the tergum of the wing-bearing segment and forms a protective, hood-like canopy over the halter. In the flexed wing, the outer squama of the alula is turned upside down above the inner squama, the latter not being affected by the movement of the wing. In many Diptera, a deep incision of the anal area of the wing membrane behind the single vannal vein sets off a proximal alar lobe distal to the outer squama of the alula.[36]
Joints
[编辑]
The various movements of the wings, especially in insects that flex their wings horizontally over their backs when at rest, demand a more complicated articular structure at the wing base than a mere hinge of the wing with the body. Each wing is attached to the body by a membranous basal area, but the articular membrane contains a number of small articular sclerites, collectively known as the pteralia. The pteralia include an anterior humeral plate at the base of the costal vein, a group of axillaries (Ax) associated with the subcostal, radial, and vannal veins, and two less definite median plates (m, m') at the base of the mediocubital area. The axillaries are specifically developed only in the wing-flexing insects, where they constitute the flexor mechanism of the wing operated by the flexor muscle arising on the pleuron. Characteristic of the wing base is also a small lobe on the anterior margin of the articular area proximal to the humeral plate, which, in the forewing of some insects, is developed into a large, flat, scale-like flap, the tegula, overlapping the base of the wing. Posteriorly, the articular membrane often forms an ample lobe between the wing and the body, and its margin is generally thickened and corrugated, giving the appearance of a ligament, the so-called axillary cord, continuous mesally with the posterior marginal scutellar fold of the tergal plate bearing the wing.[36]
The articular sclerites, or pteralia, of the wing base of the wing-flexing insects and their relations to the body and the wing veins, shown diagrammatically, are as follows:
- Humeral plates
- First Axillary
- Second Axillary
- Third Axillary
- Fourth Axillary
- Median plates (m, m')
The humeral plate is usually a small sclerite on the anterior margin of the wing base, movable and articulated with the base of the costal vein. Odonata have their humeral plates greatly enlargened,[36] with two muscles arising from the episternum inserted into the humeral plates and two from the edge of the epimeron inserted into the axillary plate.[24]
The first axillary sclerite (lAx) is the anterior hinge plate of the wing base. Its anterior part is supported on the anterior notal wing process of the tergum (ANP); its posterior part articulates with the tergal margin. The anterior end of the sclerite is generally produced as a slender arm, the apex of which (e) is always associated with the base of the subcostal vein (Sc), though it is not united with the latter. The body of the sclerite articulates laterally with the second axillary. The second axillary sclerite (2Ax) is more variable in form than the first axillary, but its mechanical relations are no less definite. It is obliquely hinged to the outer margin of the body of the first axillary, and the radial vein (R) is always flexibly attached to its anterior end (d). The second axillary presents both a dorsal and a ventral sclerotization in the wing base; its ventral surface rests upon the fulcral wing process of the pleuron. The second axillary, therefore, is the pivotal sclerite of the wing base, and it specifically manipulates the radial vein.[36]
The third axillary sclerite (3Ax) lies in the posterior part of the articular region of the wing. Its form is highly variable and often irregular, but the third axillary is the sclerite on which is inserted the flexor muscle of the wing (D). Mesally, it articulates anteriorly (f) with the posterior end of the second axillary, and posteriorly (b) with the posterior wing process of the tergum (PNP), or with a small fourth axillary when the latter is present. Distally, the third axillary is prolonged in a process always associated with the bases of the group of veins in the anal region of the wing, here termed the vannal veins (V). The third axillary, therefore, is usually the posterior hinge plate of the wing base and is the active sclerite of the flexor mechanism, which directly manipulates the vannal veins. The contraction of the flexor muscle (D) revolves the third axillary on its mesal articulations (b, f), and thereby lifts its distal arm; this movement produces the flexion of the wing. The fourth axillary sclerite is not a constant element of the wing base. When present, it is usually a small plate intervening between the third axillary and the posterior notal wing process, and is probably a detached piece of the latter.[36]
The median plates (m, m') are also sclerites that are not so definitely differentiated as specific plates as are the three principal axillaries, but they are important elements of the flexor apparatus. They lie in the median area of the wing base distal to the second and third axillaries, and are separated from each other by an oblique line (bf), which forms a prominent convex fold during flexion of the wing. The proximal plate (m) is usually attached to the distal arm of the third axillary and perhaps should be regarded as a part of the latter. The distal plate (m') is less constantly present as a distinct sclerite, and may be represented by a general sclerotization of the base of the mediocubital field of the wing. When the veins of this region are distinct at their bases, they are associated with the outer median plate.[36]
Coupling, folding, and other features
[编辑]In many insect species, the forewing and hindwing are coupled together, which improves the aerodynamic efficiency of flight. The most common coupling mechanism (e.g., Hymenoptera and Trichoptera) is a row of small hooks on the forward margin of the hindwing, or "hamuli", which lock onto the forewing, keeping them held together (hamulate coupling). In some other insect species (e.g., Mecoptera, Lepidoptera, and some Trichoptera) the jugal lobe of the forewing covers a portion of the hindwing (jugal coupling), or the margins of the forewing and hindwing overlap broadly (amplexiform coupling), or the hindwing bristles, or frenulum, hook under the retaining structure or retinalucum on the forewing.[1]:43
When at rest, the wings are held over the back in most insects, which may involve longitudinal folding of the wing membrane and sometimes also transverse folding. Folding may sometimes occur along the flexion lines. Though fold lines may be transverse, as in the hindwings of beetles and earwigs, they are normally radial to the base of the wing, allowing adjacent sections of a wing to be folded over or under each other. The commonest fold line is the jugal fold, situated just behind the third anal vein,[25] although, most Neoptera have a jugal fold just behind vein 3A on the forewings. It is sometimes also present on the hindwings. Where the anal area of the hindwing is large, as in Orthoptera and Blattodea, the whole of this part may be folded under the anterior part of the wing along a vannal fold a little posterior to the claval furrow. In addition, in Orthoptera and Blattodea, the anal area is folded like a fan along the veins, the anal veins being convex, at the crests of the folds, and the accessory veins concave. Whereas the claval furrow and jugal fold are probably homologous in different species, the vannal fold varies in position in different taxa. Folding is produced by a muscle arising on the pleuron and inserted into the third axillary sclerite in such a waythat, when it contracts, the sclerite pivots about its points of articulation with the posterior notal process and the second axillary sclerite.[24]
As a result, the distal arm of the third axillary sclerite rotates upwards and inwards, so that finally its position is completely reversed. The anal veins are articulated with this sclerite in such a way that when it moves they are carried with it and become flexed over the back of the insect. Activity of the same muscle in flight affects the power output of the wing and so it is also important in flight control. In orthopteroid insects, the elasticity of the cuticle causes the vannal area of the wing to fold along the veins. Consequently, energy is expended in unfolding this region when the wings are moved to the flight position. In general, wing extension probably results from the contraction of muscles attached to the basalar sclerite or, in some insects, to the subalar sclerite.[24]
腿
[编辑]The typical and usual segments of the insect leg are divided into the coxa, one trochanter, the femur, the tibia, the tarsus, and the pretarsus. The coxa in its more symmetrical form, has the shape of a short cylinder or truncate cone, though commonly it is ovate and may be almost spherical. The proximal end of the coxa is girdled by a submarginal basicostal suture that forms internally a ridge, or basicosta, and sets off a marginal flange, the coxomarginale, or basicoxite. The basicosta strengthens the base of the coxa and is commonly enlarged on the outer wall to give insertion to muscles; on the mesal half of the coxa, however, it is usually weak and often confluent with the coxal margin. The trochanteral muscles that take their origin in the coxa are always attached distal to the basicosta. The coxa is attached to the body by an articular membrane, the coxal corium, which surrounds its base. These two articulations are perhaps the primary dorsal and ventral articular points of the subcoxo-coxal hinge. In addition, the insect coxa has often an anterior articulation with the anterior, ventral end of the trochantin, but the trochantinal articulation does not coexist with a sternal articulation. The pleural articular surface of the coxa is borne on a mesal inflection of the coxal wall. If the coxa is movable on the pleural articulation alone, the coxal articular surface is usually inflected to a sufficient depth to give a leverage to the abductor muscles inserted on the outer rim of the coxal base. Distally the coxa bears an anterior and a posterior articulation with the trochanter. The outer wall of the coxa is often marked by a suture extending from the base to the anterior trochanteral articulation. In some insects the coxal suture falls in line with the pleural suture, and in such cases the coxa appears to be divided into two parts corresponding to the episternum and epimeron of the pleuron. The coxal suture is absent in many insects.[36]:163–164
The inflection of the coxal wall bearing the pleural articular surface divides the lateral wall of the basicoxite into a prearticular part and a postarticular part, and the two areas often appear as two marginal lobes on the base of the coxa. The posterior lobe is usually the larger and is termed the meron. The meron may be greatly enlarged by an extension distally in the posterior wall of the coxa; in the Neuroptera, Mecoptera, Trichoptera, and Lepidoptera, the meron is so large that the coxa appears to be divided into an anterior piece, the so-called "coxa genuina," and the meron, but the meron never includes the region of the posterior trochanteral articulation, and the groove delimiting it is always a part of the basicostal suture. A coxa with an enlarged meron has an appearance similar to one divided by a coxal suture falling in line with the pleural suture, but the two conditions are fundamentally quite different and should not be confused. The meron reaches the extreme of its departure from the usual condition in the Diptera. In some of the more generalized flies, as in the Tipulidae, the meron of the middle leg appears as a large lobe of the coxa projecting upward and posteriorly from the coxal base; in higher members of the order it becomes completely separated from the coxa and forms a plate of the lateral wall of the mesothorax.[36]:164
The trochanter is the basal segment of the telopodite; it is always a small segment in the insect leg, freely movable by a horizontal hinge on the coxa, but more or less fixed to the base of the femur. When movable on the femur the trochantero femoral hinge is usually vertical or oblique in a vertical plane, giving a slight movement of production and reduction at the joint, though only a reductor muscle is present. In the Odonata, both nymphs and adults, there are two trochanteral segments, but they are not movable on each other; the second contains the reductor muscle of the femur. The usual single trochanteral segment of insects, therefore, probably represents the two trochanters of other arthropods fused into one apparent segment, since it is not likely that the primary coxotrochanteral hinge has been lost from the leg. In some of the Hymenoptera a basal subdivision of the femur simulates a second trochanter, but the insertion of the reductor muscle on its base attests that it belongs to the femoral segment, since as shown in the odonate leg, the reductor has its origin in the true second trochanter.[36]:165
The femur is the third segment of the insect leg, is usually the longest and strongest part of the limb, but it varies in size from the huge hind femur of leaping Orthoptera to a very small segment such as is present in many larval forms. The volume of the femur is generally correlated with the size of the tibial muscles contained within it, but it is sometimes enlarged and modified in shape for other purposes than that of accommodating the tibial muscles. The tibia is characteristically a slender segment in adult insects, only a little shorter than the femur or the combined femur and trochanter. Its proximal end forms a more or less distinct head bent toward the femur, a device allowing the tibia to be flexed close against the under surface of the femur.[36]:165
The terms profemur, mesofemur and metafemur refer to the femora of the front, middle and hind legs of an insect, respectively.[38] Similarly protibia, mesotibia and metatibia refer to the tibiae of the front, middle and hind legs.[39]
The tarsus of insects corresponds to the penultimate segment of a generalized arthropod limb, which is the segment called the propodite in Crustacea. adult insects it is commonly subdivided into from two to five subsegments, or tarsomeres, but in the Protura, some Collembola, and most holometabolous insect larvae it preserves the primitive form of a simple segment. The subsegments of the adult insect tarsus are usually freely movable on one another by inflected connecting membranes, but the tarsus never has intrinsic muscles. The tarsus of adult pterygote insects having fewer than five subsegments is probably specialized by the loss of one or more subsegments or by a fusion of adjoining subsegments. In the tarsi of Acrididae the long basal piece is evidently composed of three united tarsomeres, leaving the fourth and the fifth. The basal tarsomere is sometimes conspicuously enlarged and is distinguished as the basitarsus. On the under surfaces of the tarsal subsegments in certain Orthoptera there are small pads, the tarsal pulvilli, or euplantulae. The tarsus is occasionally fused with the tibia in larval insects, forming a tibiotarsal segment; in some cases it appears to be eliminated or reduced to a rudiment between the tibia and the pretarsus.[36]:165–166
For the most part the femur and tibia are the longest leg segments but variations in the lengths and robustness of each segment relate to their functions. For example, gressorial and cursorial, or walking and running type insects respectively, usually have well-developed femora and tibiae on all legs, whereas jumping (saltatorial) insects such as grasshoppers have disproportionately developed metafemora and metatibiae. In aquatic beetles (Coleoptera) and bugs (Hemiptera), the tibiae and/or tarsi of one or more pairs of legs usually are modified for swimming (natatorial) with fringes of long, slender hairs. Many ground-dwelling insects, such as mole crickets (Orthoptera: Gryllotalpidae), nymphal cicadas (Hemiptera: Cicadidae), and scarab beetles (Scarabaeidae), have the tibiae of the forelegs (protibiae) enlarged and modified for digging (fossorial), whereas the forelegs of some predatory insects, such as mantispid lacewings (Neuroptera) and mantids (Mantodea), are specialized for seizing prey, or raptorial. The tibia and basal tarsomere of each hindleg of honey bees are modified for the collection and carriage of pollen.[24]:45
腹节
[编辑]The ground plan of the abdomen of an adult insect typically consists of 11–12 segments and is less strongly sclerotized than the head or thorax. Each segment of the abdomen is represented by a sclerotized tergum, sternum, and perhaps a pleurite. Terga are separated from each other and from the adjacent sterna or pleura by a membrane. Spiracles are located in the pleural area. Variation of this ground plan includes the fusion of terga or terga and sterna to form continuous dorsal or ventral shields or a conical tube. Some insects bear a sclerite in the pleural area called a laterotergite. Ventral sclerites are sometimes called laterosternites. During the embryonic stage of many insects and the postembryonic stage of primitive insects, 11 abdominal segments are present. In modern insects there is a tendency toward reduction in the number of the abdominal segments, but the primitive number of 11 is maintained during embryogenesis.Variation in abdominal segment number is considerable. If the Apterygota are considered to be indicative of the ground plan for pterygotes, confusion reigns: adult Protura have 12 segments, Collembola have 6. The orthopteran family Acrididae has 11 segments, and a fossil specimen of Zoraptera has a 10-segmented abdomen.[8]
Generally, the first seven abdominal segments of adults (the pregenital segments) are similar in structure and lack appendages. However, apterygotes (bristletails and silverfish) and many immature aquatic insects have abdominal appendages. Apterygotes possess a pair of styles; rudimentary appendages that are serially homologous with the distal part of the thoracic legs. And, mesally, one or two pairs of protrusible (or exsertile) vesicles on at least some abdominal segments. These vesicles are derived from the coxal and trochanteral endites (inner annulated lobes) of the ancestral abdominal appendages. Aquatic larvae and nymphs may have gills laterally on some to most abdominal segments.[1]:49 Of the rest of the abdominal segments consist of the reproductive and anal parts.
The anal-genital part of the abdomen, known as the terminalia, consists generally of segments 8 or 9 to the abdominal apex. Segments 8 and 9 bear the genitalia; segment 10 is visible as a complete segment in many "lower" insects but always lacks appendages; and the small segment 11 is represented by a dorsal epiproct and pair of ventral paraprocts derived from the sternum. A pair of appendages, the cerci, articulates laterally on segment 11; typically these are annulated and filamentous but have been modified (e.g. the forceps of earwigs) or reduced in different insect orders. An annulated caudal filament, the median appendix dorsalis, arises from the tip of the epiproct in apterygotes, most mayflies (Ephemeroptera), and a few fossil insects. A similar structure in nymphal stoneflies (Plecoptera) is of uncertain homology. These terminal abdominal segments have excretory and sensory functions in all insects, but in adults there is an additional reproductive function.[1]:49
外生殖器
[编辑]
The organs concerned specifically with mating and the deposition of eggs are known collectively as the external genitalia, although they may be largely internal. The components of the external genitalia of insects are very diverse in form and often have considerable taxonomic value, particularly among species that appear structurally similar in other respects. The male external genitalia have been used widely to aid in distinguishing species, whereas the female external genitalia may be simpler and less varied.
The terminalia of adult female insects include internal structures for receiving the male copulatory organ and his spermatozoa and external structures used for oviposition (egg-laying; section 5.8). Most female insects have an egg-laying tube, or ovipositor; it is absent in termites, parasitic lice, many Plecoptera, and most Ephemeroptera. Ovipositors take two forms:
- true, or appendicular, formed from appendages of abdominal segments 8 and 9;
- substitutional, composed of extensible posterior abdominal segments.
Other appendages
[编辑]内部形态
[编辑]神经系统
[编辑]The nervous system of an insect can be divided into a brain and a ventral nerve cord. The head capsule is made up of six fused segments, each with a pair of ganglia, or a cluster of nerve cells outside of the brain. The first three pairs of ganglia are fused into the brain, while the three following pairs are fused into a structure of three pairs of ganglia under the insect's esophagus, called the subesophageal ganglion.[1]:57
The thoracic segments have one ganglion on each side, which are connected into a pair, one pair per segment. This arrangement is also seen in the abdomen but only in the first eight segments. Many species of insects have reduced numbers of ganglia due to fusion or reduction.[40] Some cockroaches have just six ganglia in the abdomen, whereas the wasp Vespa crabro has only two in the thorax and three in the abdomen. Some insects, like the house fly Musca domestica, have all the body ganglia fused into a single large thoracic ganglion.
At least a few insects have nociceptors, cells that detect and transmit sensations of pain.[41] This was discovered in 2003 by studying the variation in reactions of larvae of the common fruitfly Drosophila to the touch of a heated probe and an unheated one. The larvae reacted to the touch of the heated probe with a stereotypical rolling behavior that was not exhibited when the larvae were touched by the unheated probe.[42] Although nociception has been demonstrated in insects, there is not a consensus that insects feel pain consciously.[43]
消化系统
[编辑]An insect uses its digestive system to extract nutrients and other substances from the food it consumes.[44] Most of this food is ingested in the form of macromolecules and other complex substances like proteins, polysaccharides, fats, and nucleic acids. These macromolecules must be broken down by catabolic reactions into smaller molecules like amino acids and simple sugars before being used by cells of the body for energy, growth, or reproduction. This break-down process is known as digestion. The main structure of an insect's digestive system is a long enclosed tube called the alimentary canal, which runs lengthwise through the body. The alimentary canal directs food in one direction: from the mouth to the anus. It has three sections, each of which performs a different process of digestion. In addition to the alimentary canal, insects also have paired salivary glands and salivary reservoirs. These structures usually reside in the thorax, adjacent to the foregut.[1]:70–77 The gut is where almost all of insects' digestion takes place. It can be divided into the foregut, midgut and hindgut.
前肠
[编辑]
The first section of the alimentary canal is the foregut (element 27 in numbered diagram), or stomodaeum. The foregut is lined with a cuticular lining made of chitin and proteins as protection from tough food. The foregut includes the buccal cavity (mouth), pharynx, esophagus, and Crop and proventriculus (any part may be highly modified), which both store food and signify when to continue passing onward to the midgut.[1]:70 Here, digestion starts as partially chewed food is broken down by saliva from the salivary glands. As the salivary glands produce fluid and carbohydrate-digesting enzymes (mostly amylases), strong muscles in the pharynx pump fluid into the buccal cavity, lubricating the food like the salivarium does, and helping blood feeders, and xylem and phloem feeders.
From there, the pharynx passes food to the esophagus, which could be just a simple tube passing it on to the crop and proventriculus, and then on ward to the midgut, as in most insects. Alternately, the foregut may expand into a very enlarged crop and proventriculus, or the crop could just be a diverticulum, or fluid filled structure, as in some Diptera species.[45]:30–31

The salivary glands (element 30 in numbered diagram) in an insect's mouth produce saliva. The salivary ducts lead from the glands to the reservoirs and then forward through the head to an opening called the salivarium, located behind the hypopharynx. By moving its mouthparts (element 32 in numbered diagram) the insect can mix its food with saliva. The mixture of saliva and food then travels through the salivary tubes into the mouth, where it begins to break down.[44][46] Some insects, like flies, have extra-oral digestion. Insects using extra-oral digestion expel digestive enzymes onto their food to break it down. This strategy allows insects to extract a significant proportion of the available nutrients from the food source.[45]:31
中肠
[编辑]Once food leaves the crop, it passes to the midgut (element 13 in numbered diagram), also known as the mesenteron, where the majority of digestion takes place. Microscopic projections from the midgut wall, called microvilli, increase the surface area of the wall and allow more nutrients to be absorbed; they tend to be close to the origin of the midgut. In some insects, the role of the microvilli and where they are located may vary. For example, specialized microvilli producing digestive enzymes may more likely be near the end of the midgut, and absorption near the origin or beginning of the midgut.[45]:32
后肠
[编辑]In the hindgut (element 16 in numbered diagram), or proctodaeum, undigested food particles are joined by uric acid to form fecal pellets. The rectum absorbs 90% of the water in these fecal pellets, and the dry pellet is then eliminated through the anus (element 17), completing the process of digestion. The uric acid is formed using hemolymph waste products diffused from the Malpighian tubules (element 20). It is then emptied directly into the alimentary canal, at the junction between the midgut and hindgut. The number of Malpighian tubules possessed by a given insect varies between species, ranging from only two tubules in some insects to over 100 tubules in others.[1]:71–72, 78–80
呼吸系统
[编辑]Insect respiration is accomplished without lungs. Instead, the insect respiratory system uses a system of internal tubes and sacs through which gases either diffuse or are actively pumped, delivering oxygen directly to tissues that need it via their trachea (element 8 in numbered diagram). Since oxygen is delivered directly, the circulatory system is not used to carry oxygen, and is therefore greatly reduced. The insect circulatory system has no veins or arteries, and instead consists of little more than a single, perforated dorsal tube that pulses peristaltically. Toward the thorax, the dorsal tube (element 14) divides into chambers and acts like the insect's heart. The opposite end of the dorsal tube is like the aorta of the insect circulating the hemolymph, arthropods' fluid analog of blood, inside the body cavity.[1]:61–65[47] Air is taken in through openings on the sides of the abdomen called spiracles.
There are many different patterns of gas exchange demonstrated by different groups of insects. Gas exchange patterns in insects can range from continuous and diffusive ventilation, to discontinuous gas exchange.[1]:65–68 During continuous gas exchange, oxygen is taken in and carbon dioxide is released in a continuous cycle. In discontinuous gas exchange, however, the insect takes in oxygen while it is active and small amounts of carbon dioxide are released when the insect is at rest.[48] Diffusive ventilation is simply a form of continuous gas exchange that occurs by diffusion rather than physically taking in the oxygen. Some species of insect that are submerged also have adaptations to aid in respiration. As larvae, many insects have gills that can extract oxygen dissolved in water, while others need to rise to the water surface to replenish air supplies, which may be held or trapped in special structures.[49][50]
循环系统
[编辑]Insect blood or haemolymph's main function is that of transport and it bathes the insect's body organs. Making up usually less than 25% of an insect's body weight, it transports hormones, nutrients and wastes and has a role in, osmoregulation, temperature control, immunity, storage (water, carbohydrates and fats) and skeletal function. It also plays an essential part in the moulting process.[51][52] An additional role of the haemolymph in some orders, can be that of predatory defence. It can contain unpalatable and malodourous chemicals that will act as a deterrent to predators.[1] Haemolymph contains molecules, ions and cells;[1] regulating chemical exchanges between tissues, haemolymph is encased in the insect body cavity or haemocoel.[1][53] It is transported around the body by combined heart (posterior) and aorta (anterior) pulsations, which are located dorsally just under the surface of the body.[1][51][52] It differs from vertebrate blood in that it doesn't contain any red blood cells and therefore is without high oxygen carrying capacity, and is more similar to lymph found in vertebrates.[1][53]
Body fluids enter through one-way valved ostia, which are openings situated along the length of the combined aorta and heart organ. Pumping of the haemolymph occurs by waves of peristaltic contraction, originating at the body's posterior end, pumping forwards into the dorsal vessel, out via the aorta and then into the head where it flows out into the haemocoel.[1][53] The haemolymph is circulated to the appendages unidirectionally with the aid of muscular pumps or accessory pulsatile organs usually found at the base of the antennae or wings and sometimes in the legs,[1] with pumping rates accelerating with periods of increased activity.[52] Movement of haemolymph is particularly important for thermoregulation in orders such as Odonata, Lepidoptera, Hymenoptera and Diptera.[1]
内分泌系统
[编辑]These glands are part of the endocrine system:
1. Neurosecretory cells
2. Corpora cardiaca
生殖系统
[编辑]雌性
[编辑]Female insects are able make eggs, receive and store sperm, manipulate sperm from different males, and lay eggs. Their reproductive systems are made up of a pair of ovaries, accessory glands, one or more spermathecae, and ducts connecting these parts. The ovaries make eggs and accessory glands produce the substances to help package and lay the eggs. Spermathecae store sperm for varying periods of time and, along with portions of the oviducts, can control sperm use. The ducts and spermathecae are lined with a cuticle.[8]:880
The ovaries are made up of a number of egg tubes, called ovarioles, which vary in size and number by species. The number of eggs that the insect is able to make vary by the number of ovarioles with the rate that eggs can be developed being also influenced by ovariole design. In meroistic ovaries, the eggs-to-be divide repeatedly and most of the daughter cells become helper cells for a single oocyte in the cluster. In panoistic ovaries, each egg-to-be produced by stem germ cells develops into an oocyte; there are no helper cells from the germ line. Production of eggs by panoistic ovaries tends to be slower than that by meroistic ovaries.[8]:880
Accessory glands or glandular parts of the oviducts produce a variety of substances for sperm maintenance, transport, and fertilization, as well as for protection of eggs. They can produce glue and protective substances for coating eggs or tough coverings for a batch of eggs called oothecae. Spermathecae are tubes or sacs in which sperm can be stored between the time of mating and the time an egg is fertilized. Paternity testing of insects has revealed that some, and probably many, female insects use the spermatheca and various ducts to control or bias sperm used in favor of some males over others.[8]:880
雄性
[编辑]The main component of the male reproductive system is the testis, suspended in the body cavity by tracheae and the fat body. The more primitive apterygote insects have a single testis, and in some lepidopterans the two maturing testes are secondarily fused into one structure during the later stages of larval development, although the ducts leading from them remain separate. However, most male insects have a pair of testes, inside of which are sperm tubes or follicles that are enclosed within a membranous sac. The follicles connect to the vas deferens by the vas efferens, and the two tubular vasa deferentia connect to a median ejaculatory duct that leads to the outside. A portion of the vas deferens is often enlarged to form the seminal vesicle, which stores the sperm before they are discharged into the female. The seminal vesicles have glandular linings that secrete nutrients for nourishment and maintenance of the sperm. The ejaculatory duct is derived from an invagination of the epidermal cells during development and, as a result, has a cuticular lining. The terminal portion of the ejaculatory duct may be sclerotized to form the intromittent organ, the aedeagus. The remainder of the male reproductive system is derived from embryonic mesoderm, except for the germ cells, or spermatogonia, which descend from the primordial pole cells very early during embryogenesis.[8]:885 The aedeagus can be quite pronounced or de minimis. The base of the aedeagus may be the partially sclerotized phallotheca, also called the phallosoma or theca. In some species the phallotheca contains a space, called the endosoma (internal holding pouch), into which the tip end of the aedeagus may be withdrawn (retracted). The vas deferens is sometimes drawn into (folded into) the phallotheca together with a seminal vesicle.[56][57]
Microscopic composition
[编辑]Internal of different taxa
[编辑]蜚蠊目
[编辑]Cockroaches are most common in tropical and subtropical climates. Some species are in close association with human dwellings and widely found around garbage or in the kitchen. Cockroaches are generally omnivorous with the exception of the wood-eating species such as Cryptocercus; these roaches are incapable of digesting cellulose themselves, but have symbiotic relationships with various protozoans and bacteria that digest the cellulose, allowing them to extract the nutrients. The similarity of these symbionts in the genus Cryptocercus to those in termites are such that it has been suggested that they are more closely related to termites than to other cockroaches,[58] and current research strongly supports this hypothesis of relationships.[59] All species studied so far carry the obligate mutualistic endosymbiont bacterium Blattabacterium, with the exception of Nocticola australiensis, an Australian cave dwelling species without eyes, pigment or wings, and which recent genetic studies indicates are very primitive cockroaches.[60][61]
Cockroaches, like all insects, breathe through a system of tubes called tracheae. The tracheae of insects are attached to the spiracles, excluding the head. Thus cockroaches, like all insects, are not dependent on the mouth and windpipe to breathe. The valves open when the CO2 level in the insect rises to a high level; then the CO2 diffuses out of the tracheae to the outside and fresh O2 diffuses in. Unlike in vertebrates that depend on blood for transporting O2 and CO2, the tracheal system brings the air directly to cells, the tracheal tubes branching continually like a tree until their finest divisions, tracheoles, are associated with each cell, allowing gaseous oxygen to dissolve in the cytoplasm lying across the fine cuticle lining of the tracheole. CO2 diffuses out of the cell into the tracheole. While cockroaches do not have lungs and thus do not actively breathe in the vertebrate lung manner, in some very large species the body musculature may contract rhythmically to forcibly move air out and in the spiracles; this may be considered a form of breathing.[62]
鞘翅目
[编辑]The digestive system of beetles is primarily based on plants, which they for the most part feed upon, with mostly the anterior midgut performing digestion. However, in predatory species (e.g., Carabidae) most digestion occurs in the crop by means of midgut enzymes. In Elateridae species, the predatory larvae defecate enzymes on their prey, with digestion being extraorally.[8] The alimentary canal basically comprises a short narrow pharynx, a widened expansion, the crop and a poorly developed gizzard. After there is a midgut, that varies in dimensions between species, with a large amount of cecum, with a hingut, with varying lengths. There are typically four to six Malpighian tubules.[63]
The nervous system in beetles contains all the types found in insects, varying between different species. With three thoracic and seven or eight abdominal ganglia can be distinguished to that in which all the thoracic and abdominal ganglia are fused to form a composite structure. Oxygen is obtained via a tracheal system. Air enters a series of tubes along the body through openings called spiracles, and is then taken into increasingly finer fibers.[8] Pumping movements of the body force the air through the system. Some species of diving beetles (Dytiscidae) carry a bubble of air with them whenever they dive beneath the water surface. This bubble may be held under the elytra or it may be trapped against the body using specialized hairs. The bubble usually covers one or more spiracles so the insect can breathe air from the bubble while submerged. An air bubble provides an insect with only a short-term supply of oxygen, but thanks to its unique physical properties, oxygen will diffuse into the bubble and displacing the nitrogen, called passive diffusion, however the volume of the bubble eventually diminishes and the beetle will have to return to the surface.[64]
Like other insect species, beetles have hemolymph instead of blood. The open circulatory system of the beetle is driven by a tube-like heart attached to the top inside of the thorax.
Different glands specialize for different pheromones produced for finding mates. Pheromones from species of Rutelinea are produced from epithelial cells lining the inner surface of the apical abdominal segments or amino acid based pheromones of Melolonthinae from eversible glands on the abdominal apex. Other species produce different types of pheromones. Dermestids produce esters, and species of Elateridae produce fatty-acid-derived aldehydes and acetates.[8] For means of finding a mate also, fireflies (Lampyridae) utilized modified fat body cells with transparent surfaces backed with reflective uric acid crystals to biosynthetically produce light, or bioluminescence. The light produce is highly efficient, as it is produced by oxidation of luciferin by the enzymes luciferase in the presence of ATP (adenosine triphospate) and oxygen, producing oxyluciferin, carbon dioxide, and light.[8]
A notable number of species have developed special glands that produce chemicals for deterring predators (see Defense and predation). The Ground beetle's (of Carabidae) defensive glands, located at the posterior, produce a variety of hydrocarbons, aldehydes, phenols, quinones, esters, and acids released from an opening at the end of the abdomen. While African carabid beetles (e.g., Anthia some of which used to comprise the genus Thermophilum) employ the same chemicals as ants: formic acid.[29] While Bombardier beetles have well developed, like other carabid beetles, pygidial glands that empty from the lateral edges of the intersegment membranes between the seventh and eighth abdominal segments. The gland is made of two containing chambers. The first holds hydroquinones and hydrogen peroxide, with the second holding just hydrogen peroxide plus catalases. These chemicals mix and result in an explosive ejection, forming temperatures of around 100 C, with the breakdown of hydroquinone to H2 + O2 + quinone, with the O2 propelling the excretion.[8]
Tympanal organs are hearing organs. Such an organ is generally a membrane (tympanum) stretched across a frame backed by an air sac and associated sensory neurons. In the order Coleoptera, tympanal organs have been described in at least two families.[28] Several species of the genus Cicindela in the family Cicindelidae have ears on the dorsal surface of the first abdominal segment beneath the wing; two tribes in the family Dynastinae (Scarabaeidae) have ears just beneath the pronotal shield or neck membrane. The ears of both families are to ultrasonic frequencies, with strong evidence that they function to detect the presence of bats via their ultrasonic echolocation. Even though beetles constitute a large order and live in a variety of niches, examples of hearing is surprisingly lacking in species, though it is likely that most are just undiscovered.[8]
革翅目
[编辑]The neuroendocrine system is typical of insects. There is a brain, a subesophageal ganglion, three thoracic ganglia, and six abdominal ganglia. Strong neuron connections connect the neurohemal corpora cardiaca to the brain and frontal ganglion, where the closely related median corpus allatum produces juvenile hormone III in close proximity to the neurohemal dorsal arota. The digestive system of earwigs is like all other insects, consisting of a fore-, mid-, and hindgut, but earwigs lack gastric caecae which are specialized for digestion in many species of insect. Long, slender (extratory) malpighian tubules can be found between the junction of the mid- and hind gut.[8]
The reproductive system of females consist of paired ovaries, lateral oviducts, spermatheca, and a genital chamber. The lateral ducts are where the eggs leave the body, while the spermatheca is where sperm is stored. Unlike other insects, the gonopore, or genital opening is behind the seventh abdominal segment. The ovaries are primitive in that they are polytrophic (the nurse cells and oocytes alternate along the length of the ovariole). In some species these long ovarioles branch off the lateral duct, while in others, short ovarioles appear around the duct.[8]
双翅目
[编辑]The genitalia of female flies are rotated to a varying degree from the position found in other insects. In some flies this is a temporary rotation during mating, but in others it is a permanent torsion of the organs that occurs during the pupal stage. This torsion may lead to the anus being located below the genitals, or, in the case of 360° torsion, to the sperm duct being wrapped around the gut, despite the external organs being in their usual position. When flies mate, the male initially flies on top of the female, facing in the same direction, but then turns round to face in the opposite direction. This forces the male to lie on its back in order for its genitalia to remain engaged with those of the female, or the torsion of the male genitals allows the male to mate while remaining upright. This leads to flies having more reproduction abilities than most insects and at a much quicker rate. Flies come in great populations due ir ability to mate effectively and in a short period of time especially during the mating season.[65]
The female lays her eggs as close to the food source as possible, and development is very rapid, allowing the larva to consume as much food as possible in a short period of time before transforming into the adult. The eggs hatch immediately after being laid, or the flies are ovoviviparous, with the larva hatching inside the mother.[65] Larval flies, or maggots, have no true legs, and little demarcation between the thorax and abdomen; in the more derived species, the head is not clearly distinguishable from the rest of the body. Maggots are limbless, or else have small prolegs. The eyes and antennae are reduced or absent, and the abdomen also lacks appendages such as cerci. This lack of features is an adaptation to a food-rich environment, such as within rotting organic matter, or as an endoparasite.[65] The pupae take various forms, and in some cases develop inside a silk cocoon. After emerging from the pupa, the adult fly rarely lives more than a few days, and serves mainly to reproduce and to disperse in search of new food sources.
鳞翅目
[编辑]In reproductive system of butterflies and moths, the male genitalia are complex and unclear. In females there are three types of genitalia based on the relating taxa: monotrysian, exoporian, and dytresian. In the monotrysian type there is an opening on the fused segments of the sterna 9 and 10, which act as insemination and oviposition. In the exoporian type (in Hepaloidae and Mnesarchaeoidea) there are two separate places for insemination and oviposition, both occurring on the same sterna as the monotrysian type, 9/10. In most species the genitalia are flanked by two soft lobes, although they may be specialized and sclerotized in some species for ovipositing in area such as crevices and inside plant tissue.[63] Hormones and the glands that produce them run the development of butterflies and moths as they go through their life cycle, called the endocrine system. The first insect hormone PTTH (Prothoracicotropic hormone) operates the species life cycle and diapause (see the relates section).[66] This hormone is produced by corpora allata and corpora cardiaca, where it is also stored. Some glands are specialized to perform certain task such as producing silk or producing saliva in the palpi.[1]:65, 75 While the corpora cardiaca produce PTTH, the corpora allata also produces jeuvanile hormones, and the prothorocic glands produce moulting hormones.
In the digestive system, the anterior region of the foregut has been modified to form a pharyngial sucking pump as they need it for the food they eat, which are for the most part liquids. An esophagus follows and leads to the posterior of the pharynx and in some species forms a form of crop. The midgut is short and straight, with the hindgut being longer and coiled.[63] Ancestors of lepidopteran species, stemming from Hymenoptera, had midgut ceca, although this is lost in current butterflies and moths. Instead, all the digestive enzymes other than initial digestion, are immobilized at the surface of the midgut cells. In larvae, long-necked and stalked goblet cells are found in the anterior and posterior midgut regions, respectively. In insects, the goblet cells excrete positive potassium ions, which are absorbed from leaves ingested by the larvae. Most butterflies and moths display the usual digestive cycle, however species that have a different diet require adaptations to meet these new demands.[8]:279
In the circulatory system, hemolymph, or insect blood, is used to circulate heat in a form of thermoregulation, where muscles contraction produces heat, which is transferred to the rest of the body when conditions are unfavorable.[67] In lepidopteran species, hemolymph is circulated through the veins in the wings by some form of pulsating organ, either by the heart or by the intake of air into the trachea.[1]:69 Air is taken in through spiracles along the sides of the abdomen and thorax supplying the trachea with oxygen as it goes through the lepidopteran's respiratory system. There are three different tracheae supplying oxygen diffusing oxygen throughout the species body: The dorsal, ventral, and visceral. The dorsal tracheae supply oxygen to the dorsal musculature and vessels, while the ventral tracheae supply the ventral musculature and nerve cord, and the visceral tracheae supply the guts, fat bodies, and gonads.[1]:71, 72
参见
[编辑]

- 形态学 (生物学)
- Insect physiology
- Lepidoptera morphology
- Insect ecology
- Insect flight
- 无脊椎动物
- 昆虫
- 昆虫学
- Prehistoric insect
参考文献
[编辑]- ^ 1.00 1.01 1.02 1.03 1.04 1.05 1.06 1.07 1.08 1.09 1.10 1.11 1.12 1.13 1.14 1.15 1.16 1.17 1.18 1.19 1.20 1.21 1.22 1.23 1.24 1.25 1.26 1.27 1.28 1.29 1.30 1.31 1.32 1.33 1.34 1.35 1.36 1.37 1.38 1.39 1.40 1.41 1.42 1.43 1.44 1.45 1.46 Gullan, P.J.; P.S. Cranston. The Insects: An Outline of Entomology 3. Oxford: Blackwell Publishing. 2005. ISBN 1-4051-1113-5.
- ^ O. Orkin Insect zoo. The University of Nebraska Department of Entomology. [2009-05-03]. (原始内容存档于2009-06-02).
- ^ Resh, Vincent H.; Cardé, Ring T. Encyclopedia of Insects 2nd. San DIego, CA: Academic Press. 2009: 12.
- ^ Campbell, N. A. (1996) Biology (4th edition) Benjamin Cummings, New Work. p. 69 ISBN 0-8053-1957-3
- ^ Gene Kritsky. (2002). A Survey of Entomology. iUniverse. ISBN 978-0-595-22143-1.
- ^ external morphology of Insects (PDF). [2011-03-20].
- ^ 7.0 7.1 7.2 Richards, O. W.; Davies, R.G. Imms' General Textbook of Entomology: Volume 1: Structure, Physiology and Development Volume 2: Classification and Biology. Berlin: Springer. 1977. ISBN 0-412-61390-5.
- ^ 8.00 8.01 8.02 8.03 8.04 8.05 8.06 8.07 8.08 8.09 8.10 8.11 8.12 8.13 8.14 8.15 8.16 8.17 8.18 8.19 8.20 8.21 8.22 8.23 8.24 8.25 8.26 8.27 8.28 8.29 8.30 Resh, Vincent H.; Ring T. Carde. Encyclopedia of Insects 2. U. S. A.: Academic Press. July 1, 2009. ISBN 0-12-374144-0.
- ^ 9.0 9.1 Smith, John Bernhard, Explanation of terms used in entomology Publisher: Brooklyn entomological society 1906 (May be downloaded from: https://archive.org/details/explanationofter00smit)
- ^ 10.0 10.1 Fox, Richard. External Anatomy. Lander University. 2006-10-06 [2011-03-20].
- ^ 11.0 11.1 Völkel, R; Eisner, M; Weible, K. J. Miniaturized imaging systems (PDF). Microelectronic Engineering. June 2003, 67–68 (1): 461–472. doi:10.1016/S0167-9317(03)00102-3. (原始内容 (PDF)存档于2008-10-01).
- ^ Gaten, Edward. Optics and phylogeny: is there an insight? The evolution of superposition eyes in the Decapoda (Crustacea). Contributions to Zoology. 1998, 67 (4): 223–236.
- ^ Ritchie, Alexander. Ainiktozoon loganense Scourfield, a protochordate? from the Silurian of Scotland. Alcheringa. 1985, 9 (2): 137. doi:10.1080/03115518508618961.
- ^ 14.0 14.1 Mayer, G. Structure and development of onychophoran eyes: What is the ancestral visual organ in arthropods?. Arthropod Structure and Development. 2006, 35 (4): 231–245. PMID 18089073. doi:10.1016/j.asd.2006.06.003.
- ^ 15.0 15.1 15.2 15.3 15.4 15.5 15.6 Chapman, R.F. The Insects: Structure and Function (PDF) 4th. Cambridge, UK: Cambridge University Press. 1998. ISBN 0521570484.
- ^ 16.0 16.1 Krause, A.F.; Winkler, A.; Dürr, V. Central drive and proprioceptive control of antennal movements in the walking stick insect. Journal of Physiology Paris. 2013, 107: 116–129.
- ^ 17.0 17.1 Okada, J; Toh, Y. Peripheral representation of antennal orientation by the scapal hair plate of the cockroach Periplaneta americana. Journal of Experimental Biology. 2001, 204: 4301–4309.
- ^ 18.0 18.1 Staudacher, E.; Gebhardt, M.J.; Dürr, V. Antennal movements and mechanoreception: Neurobiology of active tactile sensors. Advances in Insect Physiology. 2005, 32: 49–205.
- ^ 19.0 19.1 Servadei, A.; Zangheri, S.; Masutti, L. Entomologia generale ed applicata. CEDAM. 1972: 492–530.
- ^ 20.0 20.1 Campbell, Frank L.; Priestly, June D. Flagellar Annuli of Blattella germanica (Dictyoptera: Blattellidae).–Changes in Their Numbers and Dimensions during Postembryonic Development. Annals of the Entomological Society of America. 1970, 63: 81–88. doi:10.1093/aesa/63.1.81.
- ^ 21.0 21.1 Insect antennae. The Amateur Entomologists' Society. [2011-03-21].
- ^ 22.0 22.1 22.2 22.3 Insect Morphology. University of Minisotta (Department of Entomology). [2011-03-21]. (原始内容存档于2011-03-03).
- ^ 23.0 23.1 Kirejtshuk, A.G. Head. Beetles (Coleoptera) and coleopterologist. zin.ru. November 2002 [2011-03-21].
- ^ 24.00 24.01 24.02 24.03 24.04 24.05 24.06 24.07 24.08 24.09 24.10 24.11 24.12 24.13 24.14 24.15 24.16 24.17 24.18 24.19 24.20 24.21 Chapman, R.F. The Insects: Structure and function 4th. Cambridge, New York: Cambridge University Press. 1998. ISBN 0-521-57048-4.
- ^ 25.0 25.1 25.2 25.3 25.4 25.5 25.6 25.7 Gilliott, Cedric. Entomology 2. Springer-Verlag New York, LLC. August 1995. ISBN 0-306-44967-6.
- ^ Kapoor, V.C. C. Principles and Practices of Animal Taxonomy 1 1. Science Publishers. January 1998: 48. ISBN 1-57808-024-X.
- ^ 27.0 27.1 Mosquito biting mouthparts. allmosquitos.com. 2011 [2011-04-17].
- ^ 28.0 28.1 28.2 28.3 28.4 28.5 28.6 Scoble, MJ. The Lepidoptera: Form, function, and diversity.. Oxford Univ. Press. 1992. ISBN 978-1-4020-6242-1.
- ^ 29.0 29.1 29.2 Evans, Arthur V.; Bellamy, Charles. An Inordinate Fondness for Beetles. April 2000. ISBN 0-520-22323-3.
- ^ 30.0 30.1 Heppner, J. B. Butterflies and moths. Capinera, John L. (编). Encyclopedia of Entomology. Gale virtual reference library 4 2nd. Springer Reference. 2008: 4345. ISBN 978-1-4020-6242-1.
- ^ 31.0 31.1 Agosta, Salvatore J.; Janzen, Daniel H. Body size distributions of large Costa Rican dry forest moths and the underlying relationship between plant and pollinator morphology. Oikos. 2004, 108 (1): 183–193. doi:10.1111/j.0030-1299.2005.13504.x.
- ^ Kunte, Krushnamegh. Allometry and functional constraints on proboscis lengths in butterflies (PDF). Functional Ecology. 2007, 21 (5): 982–987 [2011-02-26]. doi:10.1111/j.1365-2435.2007.01299.x.
- ^ 33.0 33.1 Sponging. University of Minissota. [2011-04-17].
- ^ 34.0 34.1 Fly Mouthparts. School of Biological Sciences Online Learning Resources. University of Sydney. 2010-02-04 [2011-04-17].
- ^ 35.0 35.1 Meyer, John R. External Anatomy: WINGS. Department of Entomology, NC State University. 2007-01-05 [2011-03-21].
- ^ 36.00 36.01 36.02 36.03 36.04 36.05 36.06 36.07 36.08 36.09 36.10 36.11 36.12 36.13 36.14 36.15 36.16 36.17 36.18 36.19 36.20 36.21 36.22 36.23 36.24 Snodgrass, R. E. Principles of Insect Morphology. Cornell Univ Press. December 1993. ISBN 0-8014-8125-2.
- ^ 37.0 37.1 Spieth, HT. A New Method of Studying the Wing Veins of the Mayflies and Some Results Therefrom (Ephemerida) (PDF). Entomological News. 1932.
- ^ profemur, profemora - BugGuide.Net. bugguide.net. [2016-12-10].
- ^ protibia - BugGuide.Net. bugguide.net. [2016-12-10].
- ^ Schneiderman, Howard A. Discontinuous respiration in insects: role of the spiracles. Biol. Bull. 1960, 119 (3): 494–528. JSTOR 1539265. doi:10.2307/1539265.
- ^ Eisemann, WK; Jorgensen, W. K.; Merritt, D. J.; Rice, M. J.; Cribb, B. W.; Webb, P. D.; Zalucki, M. P.; et al. Do insects feel pain? — A biological view. Cellular and Molecular Life Sciences. 1984, 40 (2): 1420–1423. doi:10.1007/BF01963580.
- ^ Tracey, J; Wilson, RI; Laurent, G; Benzer, S; et al. painless, a Drosophila gene essential for nociception. Cell. 2003-04-18, 113 (2): 261–273. PMID 12705873. doi:10.1016/S0092-8674(03)00272-1.
- ^ Sømme, LS. Sentience and pain in invertebrates. Norwegian Scientific Committee for Food Safety. 2005-01-14 [2009-09-30].
- ^ 44.0 44.1 General Entomology - Digestive and Excritory system. NC state University. [2009-05-03].
- ^ 45.0 45.1 45.2 Nation, James L. 15. Insect Physiology and Biochemistry 1. CRC Press. November 2001: 496pp. ISBN 0-8493-1181-0.
- ^ Duncan, Carl D. A Contribution to The Biology of North American Vespine Wasps 1. Stanford: Stanford University Press. 1939: 24–29.
- ^ Meyer, John R. Circulatory System. NC State University: Department of Entomology, NC State University: 1. 2006-02-17 [2009-10-11].
- ^ Chown, S.L.; S.W. Nicholson. Insect Physiological Ecology. New York: Oxford University Press. 2004. ISBN 0-19-851549-9.
- ^ Richard W. Merritt, Kenneth W. Cummins, and Martin B. Berg (editors). An Introduction to the Aquatic Insects of North America 4th. Kendall Hunt Publishers. 2007. ISBN 978-0-7575-5049-2.
- ^ Merritt, RW, KW Cummins, and MB Berg. An Introduction To The Aquatic Insects Of North America. Kendall Hunt Publishing Company. 2007. ISBN 0-7575-4128-3.
- ^ 51.0 51.1 McGavin, G. C. Essential Entomology; An order by order introduction. New York: Oxford University Press. 2001.
- ^ 52.0 52.1 52.2 Triplehorn, C. A.; Johnson, N. F. Borror and DeLong's Introduction to the Study of Insects (7th). Brooks / Thomson Cole: Brooks / Thomson Cole. 2005.
- ^ 53.0 53.1 53.2 Elzinga, R.J. Fundamentals of Entomology 6th. New Jersey USA: Pearson/Prentice Hall. 2004.
- ^ Triplehorn, Charles A; Johnson, Norman F. Borror and DeLong's introduction to the study of insects. 7th. Australia: Thomson, Brooks/Cole. 2005. ISBN 9780030968358.
- ^ Gullan, P.J.; P.S. Cranston. The Insects: An Outline of Entomology 3. Oxford: Blackwell Publishing. 2005: 61–65. ISBN 1-4051-1113-5.
- ^ De Carlo; J. A. Hemipteros acuáticos y semiacuáticos. Estudio en grupos en las partes de igual función de los aparatos genitales masculinos de especies estudiadas. Revista de la Sociedad Entomológica Argentina. 1983, 42 (1/4): 149–154.
- ^ Andersen, N. Møller. Marine insects: genital morphology, phylogeny and evolution of sea skaters, genus Halobates (Hemiptera: Gerridae). Zoological Journal of the Linnean Society. 1991, 103 (1): 21–60. doi:10.1111/j.1096-3642.1991.tb00896.x.
- ^ Eggleton, P. Termites and trees: a review of recent advances in termite phylogenetics. Insectes Sociaux. 2001, 48: 187–193. doi:10.1007/pl00001766.
- ^ Lo, Nathan; Claudio Bandi; Hirofumi Watanabe; Christine Nalepa; Tiziana Beninat. Evidence for Cocladogenesis Between Diverse Dictyopteran Lineages and Their Intracellular Endosymbionts (PDF). Molecular Biology and Evolution. 2003, 20 (6): 907–913. PMID 12716997. doi:10.1093/molbev/msg097.
- ^ Leung, Chee Chee Leung. Cave may hold missing link. theage.com.au. 2007-03-22 [2013-12-07].
- ^ Lo, N; Beninati, T; Stone, F; Walker, J; Sacchi, L. Cockroaches that lack Blattabacterium endosymbionts: the phylogenetically divergent genus Nocticola. Biology Letters. 2007, 3 (3): 327–30. PMC 2464682
. PMID 17376757. doi:10.1098/rsbl.2006.0614.
- ^ Kunkel, Joseph G. How do cockroaches breathe?. The Cockroach FAQ. UMass Amherst. [2013-12-07].
- ^ 63.0 63.1 63.2 Gillot, C. Butterflies and moths. Entomology 2. 1995: 246–266 [2010-11-14]. ISBN 978-0-306-44967-3.
- ^ Schmidt-Nielsen, Knut. Insect Respiration. Animal Physiology: Adaptation and Environment 5 (illustrated). Cambridge University Press. 1997-01-15: 55 [2010-03-06]. ISBN 0-521-57098-0.
- ^ 65.0 65.1 65.2 Hoell, H.V., Doyen, J.T. & Purcell, A.H. Introduction to Insect Biology and Diversity, 2nd ed.. Oxford University Press. 1998: 493–499. ISBN 0-19-510033-6.
- ^ Williams, C. M. Physiology of insect diapause. II. Interaction between the pupal brain and prothoracic glands in the metamorphosis of the giant silkworm "Platysamia cecropia". Biol. Bull. 1947, 92: 89–180.
- ^ Lighton, J. R. B.; Lovegrove, B. G. A temperature-induced switch from diffusive to convective ventilation in the honeybee. Journal of Experimental Biology. 1990, 154: 509–516.